Guidelines for Stem Cell Research and Clinical Translation
-
The ISSCR Guidelines address the international diversity of cultural, political, legal, and ethical issues associated with stem cell research and its translation to medicine. The guidelines maintain and underscore widely shared principles in science that call for rigor, oversight, and transparency in all areas of practice. Adherence to these principles provides assurance that stem cell research is conducted with scientific and ethical integrity and that new therapies are evidence-based.
Responding to advances in science, the guidelines were updated in 2021 to encompass a broader and more expansive scope of research and clinical endeavor while maintaining the fundamental principles of the research and application. The 2021 guidelines include new recommendations to address the recent scientific advances involving embryos, stem cell-based embryo models, chimeras, organoids, and genome editing.
-
Jump to each section of the Guidelines for Stem Cell Research and Clinical Translation. Learn more.
-
Commonly sought content from multiple areas of the Guidelines, making it easier to learn of changes. Learn more.
-
Take a look at supporting papers published in Stem Cell Reports and Nature that offer insights from members of the Guidelines Steering Committee. Learn more.
Table of Contents
1. Fundamental Ethical Principles
The primary societal mission of basic biomedical research and its clinical translation is to alleviate and prevent human suffering caused by illness and injury. All such biomedical research is a collective effort.
Return to TOC | 2021 Guidelines for Stem Cell Research and Clinical Translation
The primary societal mission of basic biomedical research and its clinical translation is to alleviate and prevent human suffering caused by illness and injury. All such biomedical research is a collective effort. It depends on the public support and contributions of many individuals, including scientists, clinicians, patients and their advocates, research participants, members of industry, regulators and other governmental officials, legislators, and others. Such individuals often work across institutions, professions, and national boundaries and are governed by different social and cultural beliefs, regulatory systems, and expectations for moral conduct. Each may also be working toward different goals. When this collective effort works well, the social mission of responsible basic research and clinical translation is achieved efficiently alongside the private interests of its various contributors.
Ethical principles and guidelines help secure the basis for this collective effort together with an internationally coordinated framework to regulate research at all levels, including clinical trials and market access to proven interventions. This helps to give the public and research funding organizations confidence that generally accepted ethical boundaries will not be crossed in either basic or clinical research. Patients should be able to enroll in clinical research trusting that studies are well justified, appropriately designed and ethically sound, the risks and burdens are reasonable in relation to potential benefits, the quality and manufacturing of the experimental product fulfills the standards expected for safe human administration, and the study will collect meaningful information to support further development of the intervention. Physicians and payers need to be confident that the evidence they use to make important healthcare decisions is rigorous and unbiased. Organizations, including private firms, can invest in research and product development programs knowing that products will be promptly and fairly evaluated by regulators.
The International Society for Stem Cell Research (ISSCR) Guidelines pertain to human stem cell research, clinical translation, and related research activities. These guidelines promote an ethical, practical, appropriate, and sustainable enterprise for stem cell research and the development of cell therapies that will improve human health and should be available for patients in need. These guidelines do not supersede local laws and regulations. However, they complement existing legal frameworks and can inform the interpretation and development of laws applicable to stem cell research as well as provide guidance for research practices not covered by legislation. These guidelines build on a set of widely shared ethical principles in science, research with human subjects, and medicine (Nuremberg Code, 1949; Decleration of Helsinki of the WMA, 1964; Department of Health, and Education and Welfare, 1979; European Science Foundation, 2000; Medical Professionalism Project, 2002; Institute of Medicine, 2009; World Medical Association, 2018; Council for International Organizations of Medical Sciences, 2016). Some of the guidelines that follow are applicable to all basic research and clinical translation efforts. Others respond to challenges that are especially relevant to stem cell-based research and interventions. These include sensitivities surrounding research activities that involve the use of human embryos and gametes, irreversible risks associated with some cell-based interventions, including those that involve genome editing, the vulnerability and pressing medical needs of patients with serious illnesses and medical conditions that currently lack effective treatments, public expectations about medical advance and access, and competitiveness within this research context.
Integrity of the Research Enterprise
The primary goals of stem cell research are to advance scientific understanding, to generate evidence for addressing unmet medical and public health needs, and to develop safe and efficacious therapies for patients. This research should be overseen by qualified investigators and conducted in a manner that maintains public confidence. Research, whether basic, preclinical or clinical, must ensure that the information obtained will be trustworthy, reliable, accessible, and responsive to scientific uncertainties and priority health needs. Key processes for maintaining the integrity of the research enterprise include those for independent peer review and oversight, replication, institutional oversight, and accountability at each stage of research.
Primacy of Patient/Participant Welfare
Physicians and physician-researchers owe their primary duty of care to patients and/or research subjects. They must never excessively place vulnerable patients or research subjects at risk. Clinical testing should never allow promise for future patients to override the welfare of current research subjects. Further, human subjects should be stringently protected from procedures offering no prospect of benefit that involve greater than a minor increase over minimal risk. The application of stem cell-based interventions outside formal research settings should occur after products have been authorized by regulators and proven safe and efficacious, include long-term patient follow-up and adverse event reporting, and serve patients’ best interests. It should also ensure similar standards of product quality and safety expected for early clinical use and be conducted in authorized establishments operating under a formal regulatory framework. Promising innovative strategies should be systematically evaluated as early as possible and before application in large populations. It is a breach of professional medical ethics and responsible scientific practices to market or provide stem cell-based interventions prior to rigorous and independent expert review of safety and efficacy and appropriate regulatory approval.
Respect for Patients and Research Subjects
Researchers, clinical practitioners, and healthcare institutions should empower potential human research participants (human subjects) to exercise valid informed consent where they have adequate decision-making capacity. Patients, whether in research or care settings, must be offered accurate information about risks and the current state of evidence for novel stem cell-based interventions. Where individuals lack such capacity, surrogate consent should be obtained from lawfully authorized representatives.
Transparency
Researchers should promote the timely exchange of accurate scientific information to other interested parties. Researchers should communicate with various public groups, such as patient communities and individuals from the emerging do-it-yourself biology movement, to respond to their reasonable requests for relevant and needed information, and they should convey the scientific state of the art, including uncertainty about the safety, reliability, or efficacy of potential applications. Researchers and sponsors should promote open and prompt sharing of ideas, methods, data, and materials by publishing, in a timely manner, positive and negative results.
Social and Distributive Justice
Fairness demands that the benefits of clinical translation efforts should be distributed justly and globally, with particular emphasis on addressing unmet medical and public health needs. To that end, the scientific community is encouraged to work with private and public funders to emphasize addressing unmet needs by helping to identify promising areas of research, development, and application.
Social justice considerations include challenges due to structural injustices, such as socioeconomic inequalities, extant discriminatory practices, and histories of exclusion and marginalization. Advantaged populations should make efforts to share any benefits of research with disadvantaged populations. This would include ‘capacity building,’ both training and establishment of facilities, which gives benefit in the longer term. There should also be appropriate sharing of the burdens with disadvantaged populations. Trials should strive to enroll populations that reflect diversity such as age, sex, gender identity, and ethnicity. Risks and burdens associated with clinical translation should not be borne by populations that are unlikely to benefit from the knowledge produced in these efforts. The scientific community is encouraged to work with governments and industry to develop mechanisms to reduce the cost of clinical applications.
Generally, healthcare delivery systems, governments, insurance providers, and patients should not bear the financial costs of proving the safety and efficacy of experimental stem cell-based interventions. However, in some cases these parties may choose to fund clinical development, for example if there is an unmet medical need and insufficient investment from the commercial sector. Where there is a clear and substantial commercial potential for a product, the costs of testing safety and efficacy should be met by the investors. Developers should endeavor to reduce the cost of new products to make them accessible to as many patients as possible.
Return to TOC | 2021 Guidelines for Stem Cell Research and Clinical Translation
2. Laboratory-based Human Embryonic Stem Cell Research, Embryo Research, and Related Research Activities
Stem cell and embryo research show great promise for advancing our understanding of human development and disease, including research to address issues pertinent to the earliest stages of human development
Return to TOC | 2021 Guidelines for Stem Cell Research and Clinical Translation
Stem cell and embryo research show great promise for advancing our understanding of human development and disease, including research to address issues pertinent to the earliest stages of human development, such as: the causes of miscarriage; epigenetic, genetic and chromosomal disorders; and human reproduction. Furthermore, the derivation of some types of stem cell lines necessitates the use of human embryos.
Scientific research on and with human embryos and embryonic stem cell lines in culture is viewed as ethically permissible in many countries when performed under rigorous scientific and ethical oversight. This is consistent with policy statements of other organizations, most notably, the American Society for Reproductive Medicine (Ethics in Embryo Research Task Force and Ethics Committee of the American Society for Reproductive Medicine, 2020), the European Society of Human Reproduction and Embryology (ESHRE Taskforce on Ethics and Law, 2001), the American College of Obstetricians and Gynecologists (2006) and the United Kingdom (UK) Human Fertilisation and Embryology Authority (2019). Creating embryos for research, which is permitted in relatively few jurisdictions, is required to develop and ensure both standard and novel methods involving IVF (including the use of mitochondrial replacement techniques, in vitro derived gametes, etc.) are safe, efficient, and effective as well as to give information about the first steps of human development.
This section of the Guidelines pertains to:
The banking, derivation, distribution, and preclinical use of human pluripotent stem cells, including human embryonic stem cells (hESCs).
The procurement of human embryos, gametes, and somatic cells for stem cell research and in vitro embryo studies not explicitly entailing stem cell derivation.
The transfer of human pluripotent stem cells into animal host embryos in vitro.
Generation of stem cell-based models of human development.
Animal research that involves the transfer of human stem cells or their direct derivatives into animal hosts.
Institutions and researchers conducting basic research with these human cells and tissues should follow the guidelines insofar as they pertain to the categories of review discussed below.
-
Oversight
Recommendation 2.1.1: All research that (a) involves preimplantation stages of human development, in vitro human embryo culture, derivation of new embryo-derived cells or lines, integrated stem cell-based embryo models, or (b) entails the production of human gametes in vitro when such gametes are tested by fertilization or used for the creation of embryos, shall be subject to review, approval, and ongoing monitoring, as appropriate, through a specialized oversight process capable of evaluating the unique aspects of the science and the associated ethical issues (see below).
The specialized scientific and ethics oversight process encompasses the review of human embryo and related stem cell research. The process can be performed at the institutional, local, regional, national, or international level or by some coordinated combination of those elements and need not be served by a single, specific committee, so long as the oversight process as a whole occurs effectively, impartially, and rigorously. Provided appropriate expertise is available to ensure that the scientific, ethical, and legal aspects of the research can be rigorously evaluated, the specialized oversight can occur through preexisting institutional review processes that assess the participation of human subjects in research, the procurement of human tissues in and for research, or biosafety and ethical issues associated with research. For example, existing review bodies such as the Embryonic Stem Cell Research Oversight (ESCRO; Institute of Medicine and National Research Council, 2005), Stem Cell Research Oversight (SCRO; ISSCR Guidelines, 2006), or Embryo Research Oversight (EMRO; ISSCR Guidelines 2016) committees in the U.S., or the UK HFEA and regional ethics committees (RECs), are well positioned to perform review and oversight of embryo and related research. A single rather than redundant review is preferable as long as this is thorough and capable of addressing any uniquely sensitive elements of human embryo research and hESC research.
Recommendation 2.1.2: The specialized scientific and ethics oversight process must include an assessment of the scientific rationale and merit of research proposals, the relevant expertise of the researchers, and the ethical permissibility and justification for the research as discussed below.
Scientific rationale and merit of the proposal: Research involving human embryonic cells or human embryos and gametes requires that scientific goals and methods be scrutinized to ensure scientific rigor. Adequate and appropriate scientific justification for performing the research using the specified materials is required.
Relevant expertise of researchers: Appropriate expertise and training of the researchers to perform the stated experiments must be ascertained in order to ensure the appropriate use of research materials. For the derivation of new human embryo-derived cell lines, the formation of human embryo models from stem cells, or experiments that involve the use of human embryos, relevant expertise would include prior experience with embryo culture and stem cell derivation in animal systems and competence in the culture and maintenance of human embryonic stem cells. Researchers performing derivations of embryo-derived cell lines should have a detailed, documented plan for characterization, storage, banking, and distribution of new lines.
Ethical permissibility and justification: Research goals must be assessed within an ethical framework to ensure that research proceeds in a transparent and responsible manner. The project proposal should include a discussion of alternative methods and provide a rationale for performing the experiments in a human rather than animal model system, for the proposed methodology, and if the studies involve preimplantation human embryos, a justification for the anticipated numbers to be used.
Recommendation 2.1.3: The committee or body conducting the specialized scientific and ethics oversight process is responsible for (a) advising researchers on the categorization of research (see Recommendation 2.2), (b) determining whether a research proposal constitutes permissible or non-permissible research, (c) monitoring and periodically reviewing ongoing research, and (d) overseeing the provenance of the human pluripotent stem cell lines used in Category 2 Research (see section 2.2.2).
The responsible committee or entity should interpret these guidelines, define research practices, and monitor compliance. Researchers are encouraged to consult the committee or entity for advice on how to determine whether research is exempt as Category 1A (see 2.2.1)
Composition of Research Review and Oversight Bodies
Recommendation 2.1.4: The specialized scientific and ethics oversight process should be conducted by qualified scientists, ethicists, legal and regulatory experts, and community members who are not directly engaged in the research under consideration. The oversight process must include participants with the following perspectives:
Scientists and/or physicians with relevant expertise, including representation from scientists that are not directly engaged in the research under consideration. Relevant expertise includes areas of stem cell biology, assisted reproduction, developmental biology, and clinical medicine.
Ethicists with the ability to interpret the ethical justifications for, and implications of, the research under consideration.
Those familiar with relevant local policies and statutes governing the research.
Community members, unaffiliated with the institution where the research is conducted through employment, who are impartial and reasonably familiar with the views and needs of patients and patient communities who could be benefited by stem cell research, and community standards.
Additional members with relevant expertise not already represented on the oversight body should be included as required, for example to cover research involving human genetics, physiology, molecular biology, etc.
The policies and regulations of each country or jurisdiction will determine whether the specialized scientific and ethics oversight process will be performed by internal or external bodies at the institutional or national level. Participants in the specialized scientific and ethics oversight process should be selected based on their relevant area-specific expertise (e.g., scientific, clinical, ethics, research policy). Those engaged in the oversight process must be cognizant of potential financial and non-financial conflicts of interest that might compromise the integrity of the review. Such conflicts must be disclosed, evaluated, and minimized, or eliminated as much as possible.
-
Recommendation 2.2: To ensure that human embryo and related stem cell research is proceeding with due consideration, to ensure consistency of research practices among scientists globally, and to specify the types of scientific projects that should be subject to review, the research review and oversight process should use the three categories described in this section.
2.2.1 Category 1
2.2.1A: Category 1A. Research determined to be exempt from a specialized scientific and ethics oversight process after being assessed by the appropriate existing mandates and committees for laboratory research. Category 1A research includes the following activities:
Research with human pluripotent stem cell lines that is confined to cell culture and involves routine research practices, such as differentiation into tissue-specific cell types.
Research that entails the reprogramming of human somatic cells to pluripotency (for example, the generation of induced pluripotent stem cells).
Research that entails the use of human fetal tissue and cells, only if these cells and tissues have been procured according to the recommendations under section 2.3 below.
Research on stem cell culture systems that model specific stages of development or specific anatomic structures rather than the continuous development of an intact embryo or fetus. These would include but are not restricted to models of amnion formation, neural tube development, development of primordial germ cells, placental structures, 2D or 3D models of gastrulation or post-gastrulation events, and in vitro stem cell-derived organoids in culture that recapitulate most aspects of organ function, but not those that fall under subsequent categories.
The transplantation of human stem cells, their derivatives, or other human cells into postnatal animal hosts (see Recommendation 2.2.1.1).
Organoid Research
At this time, there is no biological evidence to suggest any issues of concern, such as consciousness or pain perception with organoids corresponding to CNS tissues, that would warrant review through the specialized oversight process. However, researchers should be aware of any ethical issues that may arise in the future as organoid models become more complex through long-term maturation or through the assembly of multiple organoids (Hyun et al. 2020).
2.2.1B: Category 1B. Research that is reportable to the entity or body responsible for the specialized scientific and ethics oversight process, but not normally subject to further or ongoing review, at the discretion of the entity responsible for the oversight process and subject to regulations and policies in the jurisdiction. Category 1B research includes the following activities:
Research that entails the in vitro formation of human stem cell-based embryo models that are not intended to represent the integrated development of the entire embryo including its extraembryonic membranes.
Chimeric embryo research in which human pluripotent stem cells are transferred into non-human, mammalian embryos and cultured in vitro for the minimum time necessary to achieve the scientific objective without gestation.
Research on in vitro gametogenesis from human cells including genetically modified pluripotent stem cells, which does not involve attempts at fertilization and the generation of embryos.
Scientists pursuing Category 1A and B research are recommended to consult with appropriate institutional review committees or the committee or body responsible for the specialized scientific and ethics review process (see Recommendation 2.1.3) to determine the categorization of new research proposals. Committees with the jurisdiction over the relevant research should oversee the provenance of cells, tissues and derived human pluripotent stem cell lines to ensure that procurement and derivation is deemed acceptable according to the principles outlined in this document (see Section 2.3 and Section 2.4) and is in compliance with rigorous scientific, legal, and ethical standards.
Category 1B covers in vitro chimeric embryo research and in vitro gametogenesis for which there is no intent to generate a human embryo or fetus. Researchers are encouraged when possible to report on existing or planned in vitro experiments to the committee responsible for the specialized scientific and ethics oversight process to help identify cases that may warrant full review in the future.
Studies Transplanting Human Stem Cells or Their Direct Derivatives into the Central Nervous Systems of Animals
Recommendation 2.2.1.1: Research involving the transfer of human stem cells or their direct neural and/or glial derivatives into the central nervous systems of postnatal animal hosts requires review by institutional animal research oversight committees supplemented by reviewer expertise in stem cell or developmental biology. (ISSCR Guidelines, 2006; Academy of Medical Sciences, 2011). Such oversight should weigh the potential benefits of the research and should utilize available baseline non-human animal data grounded in rigorous scientific knowledge or reasonable inferences and involve a diligent application of animal welfare principles.
Institutions should determine whether research involving human cells that have the capacity to integrate into the nervous systems of laboratory animals requires supplementation of the pre-existing animal research review process with scientists and ethicists that have relevant topic-specific expertise.
To assist review and oversight of stem cell-based human-to-non-human animal research, the ISSCR has provided an advisory report that guides reviewers through a series of considerations not typically covered by institutional animal research committees, but that are relevant for review (Hyun et al., 2021). Past experiences with genetically altered laboratory animals have shown that reasonable caution might be warranted if changes carry the potential to produce new defects and deficits. Current best practices dictate that research involving modified animals must involve the following:
the establishment of baseline animal data;
ongoing data collection during research concerning any deviation from the norms of species-typical animals;
the use of small pilot studies to ascertain any changes in the welfare of modified animals; and
ongoing monitoring and reporting to animal research oversight committees authorized to decide the need for real-time changes in protocols and, if necessary, the withdrawal of animal subjects.
Research that may result in the presence of human gametes and their precursors in the gonads of laboratory animals is of not of significant ethical concern per se, as long as the animals are not allowed to breed (see Category 3 below).
Reviewers and investigators should follow the proposed ethical standards presented in the 2020 white paper and Appendix 1, while exercising appropriate judgment in individual situations. Research involving animals should also generally comply with the principles of the 3Rs (see: www.nc3rs.org.uk) and follow the ‘ARRIVE guidelines’ (Percie du Sert et. al 2020).
2.2.2 Category 2
2.2.2 Category 2. Forms of research with embryos, certain chimeras, and stem cell-based embryo models that are permissible only after review and approval through a specialized scientific and ethics review process. A comprehensive review should be coordinated with other relevant oversight, such as that provided by human subjects review committees, in vitro fertilization (IVF) clinic oversight bodies, and animal research review processes and the research should comply with local law and policy. All such research should have a compelling scientific rationale and necessitate the use of these materials rather than employ alternative models. The research should use the minimum number of embryos necessary to achieve the scientific objective. Forms of research requiring comprehensive review by a specialized review process encompass the following activities:
Procurement and use of IVF human embryos for research in vitro.
Procurement of human gametes to create research embryos in vitro.
Research that generates human gametes from any progenitor cell type in vitro, when this entails performing studies of fertilization that produce human zygotes and embryos. The gametes may be derived from human pluripotent stem cells, oogonia, or spermatogonial stem cells that have been maintained in vitro, and they may be genetically modified or not. Any human embryos obtained from such gametes must only be studied in vitro, or be used to derive stem cell lines, such as embryonic stem cells.
Research involving the genetic alteration of human embryos or gametes used to make embryos in vitro.
Derivation of new cell lines from human embryos (not confined to pluripotent cell lines).
Research involving the in vitro culture of human embryos where embryos are maintained in culture until the formation of the primitive streak or 14 days from fertilization, whichever occurs first.
Generation of stem cell-based embryo models that represent the integrated development of the entire embryo including its extraembryonic membranes. These integrated stem cell-based embryo models should be maintained in culture for the minimum time necessary to achieve the scientific objective.
Research aimed at generating human totipotent cells that have the potential to sustain embryonic development in vitro.
Chimera research in which human pluripotent stem cells or their derivatives with broad potential are introduced into a) a non-human embryo or fetus in utero or b) a non-human embryo in vitro followed by transfer into a non-human uterus. Such experiments – if they are scientifically justified for the use of non-human primates above all other laboratory species – must exclude great and lesser ape species hosts (i.e., chimpanzees, gorillas, orangutans, bonobos, gibbons, and siamangs), as apes are prohibited from being used for invasive research in most parts of the world.
Transferring human embryos to a human uterus following mitochondrial replacement.
Culture of Human Embryos Beyond formation of the primitive streak or 14 Days
It is currently not technically feasible to culture human embryos beyond formation of a primitive streak or 14 days post-fertilization. However, culture systems are evolving, making this a possibility in the near future. Understanding the primitive streak, early germ layer development and primordial germ cell formation in humans is crucial to improve our understanding of and interventions for infertility, in vitro fertilization, pregnancy loss, and developmental disorders that occur or originate soon after implantation. Research using embryos is also crucial to validate integrated stem cell-based embryo models, which in the future may provide a more practical alternative to understanding some aspects of early human development.
Recommendation 2.2.2.1: Given advancements in human embryo culture, and the potential for such research to yield beneficial knowledge that promotes human health and well-being, the ISSCR calls for national academies of science, academic societies, funders, and regulators to lead public conversations touching on the scientific significance as well as the societal and ethical issues raised by allowing such research. Should broad public support be achieved within a jurisdiction, and if local policies and regulations permit, a specialized scientific and ethical oversight process could weigh whether the scientific objectives necessitate and justify the time in culture beyond 14 days, ensuring that only a minimal number of embryos are used to achieve the research objectives.
Human-Animal Chimeric Embryo Research
Recommendation 2.2.2.2: Chimeric embryo and in utero research described in ‘Category 2, i’ (see above) should proceed for the minimum time necessary to achieve the scientific aim. This research must proceed incrementally, stopping at well-defined timepoints to assess the degree and scope of chimerism during development before proceeding to full gestation, if full gestation is among the well-justified goals of the research. To avoid unpredictable and widespread chimerism, researchers should endeavor to use targeted chimerism strategies to limit chimerism to a particular organ system or region of the gestating chimeric animal.
Techniques such as blastocyst complementation, whereby a specific cell type or organ is effectively deleted as the host embryo develops, can lead to a specific cell type or organ being replaced entirely by derivatives from the donor-derived pluripotent stem cells. By itself this targeted chimerism may not prevent contributions elsewhere in the chimera, thus the need for an incremental approach. Nonetheless, if the host cells have an advantage over the donor cells, such as even a slightly faster rate of cell replication, then the donor cells will be disadvantaged and effectively selected against, leading to a little or no contribution outside the organ of choice.
As a general principle, non-human primate species should only be used when all other species, more distant in evolution from humans, are inadequate for the scientifically well justified research question being pursued. Appropriate research aims include understanding human development, understanding species barriers to chimerism, and treating disease. Any research involving non-human primates must utilize common laboratory species that are widely used in biomedical research (which excludes apes). Trained veterinary staff specializing in the care of non-human primates must be closely involved in the review and oversight of studies involving the transfer of human stem cells and their derivatives into nonhuman primate hosts.
Mitochondrial Replacement Techniques
Recommendation 2.2.2.3: Further research should be undertaken to refine and assess the safety and efficacy of Mitochondrial Replacement Techniques (MRT), including minimizing a) the risk of mitochondrial carryover and b) disruptions to the interaction between mitochondrial and nuclear genomes. In addition, further research on polar body transfer techniques and the use of mitophagy or genome editing is needed to reduce or eliminate pathogenic mitochondrial DNA. Such research should be subject to review by a specialized oversight process as Category 2 Research (Section 2.1.5.2).
MRT is being explored to prevent the transmission of serious mitochondrial DNA-based diseases in at-risk pregnancies (see Section 3.4.8). MRT most commonly involves transferring the nuclear DNA from the prospective mother’s oocyte or fertilized oocyte (pronuclear stage) to that of a mitochondrial donor from which the nuclear DNA has been removed [maternal spindle transfer (MST) or pronuclear transfer (PNT), respectively]. The mitochondrial donor is selected to be free of known pathogenic mutations. Input from clinicians and scientists with relevant expertise in mitochondrial and embryo biology should augment the review process for evaluating clinical protocols that entail uterine transfer of human embryos for the purpose of human reproduction.
2.2.3 Category 3
2.2.3A Category 3A. Research activities currently not permitted. Research under this category should not be pursued at this time because the approaches are currently unsafe or raise unresolved ethical issues. There may be valid reasons for undertaking the research in the future, but this should not proceed until the safety and ethical issues are resolved. Such research includes:
Research in which human embryos that have undergone modification to their nuclear genome are transferred into or gestated in a human uterus. Genome-modified human embryos include human embryos with engineered alterations to their nuclear DNA and embryos generated from a human gamete that has had its nuclear DNA modified, when such modifications could be inherited through the germline. While there are valid reasons for pursuing this line of research, which may include situations where correcting a deleterious gene variant is the only way that prospective parents may have a genetically-related child (National Academy of Medicine, National Academy of Sciences, and the Royal Society, 2020), conduct of such research will be dependent on appropriate policies, regulations, and oversight.
Research in which human embryos that have undergone editing to their mitochondrial genome are transferred into or gestated in a human uterus, as current knowledge of such interventions is inadequate to ensure safety.
The use of human gametes differentiated from human stem cells for the purposes of fertilization and human reproduction. If the safety, policy, and regulatory issues are resolved, this approach may be desirable, for example in cases where treatment of childhood cancer has led to infertility, or as a route to heritable genome editing as indicated in (a) above.
2.2.3B Category 3B: Prohibited research activities. Research under this category should not be pursued because of broad international consensus that such experiments lack a compelling scientific rationale or are widely considered to be unethical. Such research includes:
Transfer of human stem cell-based embryo models to the uterus of either a human or animal host.
Research in which human embryos produced by reprogramming of nuclei are implanted into a human or animal uterus (often referred to as human reproductive cloning).
Research in which animal chimeras incorporating human cells with the potential to form human gametes are bred to each other.
Transfer of chimeric embryos mixing animal and human cells (whether predominantly animal or human) to the uterus of a human or great or lesser ape (i.e., chimpanzees, gorillas, orangutans, bonobos, gibbons, and siamangs).
Transfer of a human embryo(s), irrespective of its origins, to an animal uterus.
Emerging Categories of Embryo Research That Merit Close Review: Heritable Genome Editing
Recommendation 2.2.3.1: Until there is further scientific clarity regarding how to achieve desired genetic alterations, additional evidence for safety, and wider discussion and consensus on ethics (i.e., whether it should be done and, if so, under which circumstances), any attempt to edit the mitochondrial genome or modify the nuclear genome of human embryos for the purpose of human reproduction is premature and should not be permitted at this time (see Section 2.2.3A, Category 3A, a).
Preclinical research that entails modifying the nuclear genomes of gametes, zygotes, and human embryos may be permissible under a rigorous specialized oversight process (Category 2). Such research promises to enhance fundamental knowledge and is essential to inform deliberations about the potential safety and use of nuclear or mitochondrial DNA genome editing in strategies aimed at preventing the transmission of serious genetic disorders.
Scientists currently lack an adequate understanding of the fidelity and precision of techniques for genome editing of human embryos, as well as a full appreciation of the safety, ethics, and potential long-term risks and benefits to individuals born following such a process. This is described in more detail in the recent report, Heritable Human Genome Editing, from the International Commission on the Clinical Use of Human Genome Editing (National Academy of Medicine, National Academy of Sciences, and the Royal Society, 2020), which suggests a responsible translational pathway in certain circumstances, albeit one that cannot currently be met. Of note, the focus of this report was on developing a responsible translational pathway; it did not include a broad look at the societal and ethical issues, as these were outside its task. Such issues have been considered elsewhere (e.g. by the Nuffield Council on Bioethics). The forthcoming report from the WHO Advisory Committee on Developing Global Standards for Governance and Oversight of Human Genome Editing will bear in mind societal views and ethical principles, but this will focus on mechanisms of governance.
Basic and preclinical research is needed to minimize the potential harms resulting from intended and unintended edits, which could be passed to future generations, as well as direct or indirect effects of the editing process that could affect embryo viability or developmental potential.
-
The procurement of human gametes, embryos, fetal tissues, and somatic cells is integral to the conduct of human embryo and stem cell research. Human biological materials must be procured in accordance with generally accepted principles of research ethics and laws and policies in their respective jurisdictions.
2.3.1 Review Process for the Procurement of Human Cells and Tissues
Recommendation 2.3.1. The review process for the procurement of human cells and tissues should be predicated on the source of the material and its intended use as described in the three tiers below.
Tier 1. Banked and historical cell lines. The procurement of cell lines from repositories or banks is permissible if the materials have been deposited and distributed consistent with the original consent for use of the donated human cells and tissues and these guidelines (see Section 2.4, Derivation, Banking and Distribution of Human Stem Cell Lines) and the standards at the time (Sugarman et al. 2008). Toward this end, the repositories or banks should require certification from depositors confirming the ethical provenance of such cells, including consent and ethics approvals. The use of historical cell lines from pathological specimens, such as HeLa, are permissible for use in stem cell research that is otherwise compliant with these guidelines. Likewise, stem cell lines procured from vendors are permissible for stem cell research, provided the vendor generated and distributed the stem cell lines in a manner consistent with the original donor consent and contemporaneous ethical and regulatory standards. The procurement of Tier 1 cell lines should not be used for reproductive purposes.
Tier 2. Fresh human somatic cells and tissues. The procurement of fresh human somatic cells and tissues for stem cell research purposes should be reviewed by existing research review committees bolstered by stem cell specific expertise in accordance with generally accepted principles of research ethics and laws and regulations in the respective jurisdiction and these guidelines (see Sections 2.3.2 and 2.3.3).
Tier 3. Gametes and embryos. The procurement of human gametes and embryos that are destined for use in human embryo research and stem cell research must be reviewed through the specialized oversight process and existing research review committees in accordance with generally accepted principles of research ethics and laws and regulations in the respective jurisdiction and these guidelines (see Sections 2.3.2 and 2.3.3).
Review by a specialized oversight process (Tier 3) or existing research review committee bolstered by stem cell-specific expertise must ensure that vulnerable populations are not exploited due to their dependent status or any compromised ability to offer voluntary consent and that there are no undue inducements or other undue influences for the provision of human cells and tissues.
2.3.2 Informed Consent for the Donation of Human Cells and Tissues
Recommendation 2.3.2.1: Embryos, fetal tissue, and other cells and tissues should be used in research only if voluntary informed consent was obtained from the donors before the research commences. The informed consent process should be robust and document the prospect of therapeutic and commercial applications as well as the potential research uses, such as the creation of hESCs, iPSCs, other immortalized cell lines, embryos, and gametes. In the case of fetal tissue, consent from the woman donating the tissue is sufficient. In the case of embryos made with donor gametes, this consent should be obtained from the gamete donors and the party(ies) with authorization to donate the embryo.
Most patients and research subjects may donate cells or tissues with broad consent to a range of future uses; however, the broad consent does not apply to use of donated cells and tissues for reproductive purposes. The consent may be obtained at the time of tissue collection or with a re-contact for additional consent to use donated cells and tissues for reproductive purposes.
In the case that human cells and tissues are procured from a minor or adult that lacks the decision-making capacity to provide informed consent, consent must be provided by a parent, legal guardian, or other legally authorized person. Whenever feasible, the assent of the minor or decisionally incapacitated adult is also strongly encouraged.
Empirical research has shown that informed consent is most effective as a dynamic, interactive, and evolving process as opposed to a static, one-time disclosure event (Flory and Emanuel, 2004). The informed consent document alone can never take the place of a meaningful dialogue between the person obtaining consent and the donors of human cells and tissues. The informed consent process can be enhanced in the following ways:
Whenever possible, the person conducting the informed consent dialogue should have no vested interest in the research protocol. If members of the research team participate in the informed consent process, their role and any other potential conflicts of interest must be disclosed, and care taken to ensure that information is provided in a transparent, accurate, unbiased manner.
The person conducting the informed consent process should provide ample opportunities for cell and tissue donors to ask questions and discuss their involvement in the research protocol.
Counseling services should be made available upon request to any potential providers of human cells and tissues prior to procurement.
Consent processes and documents should be revised in light of new research on informed consent for all types of human biological materials procurement and where relevant, ongoing studies of the long-term risks associated with oocyte retrieval.
Separating Research Consent from Treatment
Recommendation 2.3.2.2: Informed consent for research use must be distinct from informed consent for clinical treatment.
Decisions related to the provision of gametes or the creation of embryos for fertility treatment should be a voluntary choice that is free from undue influence by researchers who propose to use these cells in research. During the course of clinical treatment, researchers may not request that members of the fertility treatment team generate more embryos or harvest more oocytes than necessary for the patient’s optimal fertility treatment. Wherever possible, the treating infertility clinician should not be the investigator who is proposing to perform research on the procured materials.
Consistent with fetal tissue research guidelines issued by the Network of European CNS Transplantation and Restoration (NECTAR) and U.S. regulations, a woman’s decision to terminate a pregnancy must not be influenced by the possible research use of her fetus’ tissues (Boer, 1994; OHRP, 1993). Informed consent for fetal tissue procurement and research should be obtained from the woman only after her decision to legally terminate her pregnancy but before the abortion procedure, or after a spontaneous abortion. Medical procedures must not put the woman at any increased risk solely to facilitate the research use of donated fetal tissues. Clinicians obtaining informed consent and clinics at which informed consent is sought may not profit from the procurement of fetal tissues for research.
Review of Cell and Tissue Collection for Embryo and Stem Cell Research
Recommendation 2.3.2.3: Review of procurement protocols must ensure that cell and tissue donors are adequately informed about the specific aspects of their voluntary research participation.
Researchers should exercise care in seeking and obtaining informed consent from prospective donors. The informed consent process should take into account language barriers, the educational level and reading comprehension level of the research subjects, and any other impediments to good communication. Empirical research has shown that comprehension during the informed consent process improves with the use of interactive methods interaction (Flory and Emanuel, 2004). To facilitate the adoption of adequate and uniform standards of informed consent for the procurement of cells and tissues for research, the ISSCR provides template documents that can be downloaded and customized to specific protocols (Appendix 2). These sample documents will need to be customized for use in specific research studies and conform to local laws and policies.
If pluripotent stem cells are to be derived from procured cells or tissues, the informed consent document and discussion should cover information that addresses key aspects of human stem cell research, including but not limited to the fact that an immortal stem cell line could be established that is a partial or full genetic match to the cell or tissue donor and that the stem cell line could be shared with other researchers outside the institution and jurisdiction for other research purposes that may not be fully anticipated at this time. For a list of informed consent discussion points, see Appendix 3.
Incidental Findings
Recommendation 2.3.2.4: Researchers should develop a policy that states whether and how incidental findings will be provided to cell and tissue donors. This policy must be explained during the informed consent process. Cell and tissue donors should be able to choose whether they wish to receive incidental findings, if any. Reporting findings with relevance to public health may be required by law in certain jurisdictions.
During the course of research with human stem cell lines, particularly lines derived from somatic cells, investigators may discover information that may be of importance to cell and tissue donors, such as BRCA1/2 mutations. Because the net harms and benefits of disclosing incidental findings to cell and tissue donors are presently unclear, a single approach to managing incidental findings may not be appropriate across all studies and jurisdictions. When studies include a plan to disclose incidental findings to research subjects, researchers must offer a practical and adequately resourced feedback mechanism that involves donors’ physicians and, where possible, the verification of any discovered incidental findings.
For a given sample, secondary researchers should adhere to the incidental findings policies that were developed by the primary researchers (or others collecting cells and tissues) and disclosed to donors during the informed consent process.
If re-contact is required, instructions on how to report incidental findings (report to provider, researcher, institution, physician, etc.) should be specified in the material transfer agreement. Re-contact is a matter for primary research sites to manage. However, secondary researchers should be aware of the incidental findings policies of either of these responsible parties.
Successful implementation of a policy on incidental findings depends crucially on the traceability of cell line distribution. Therefore, all providers and recipients should ensure that cell lines are used under strict compliance with material transfer agreements and the regulations in the informed consent process.
Consent for De-identified Cells and Tissues
Recommendation 2.3.2.5: Researchers are encouraged to discuss the potential for genomic sequencing to connect de-identified cells and tissues to donors and their relatives during the informed consent process for the donation.
Cells and tissues donated for research are often de-identified to protect the privacy of donors. Due to advances in genomic sequencing, it may be possible for researchers to connect de-identified cell and tissue samples with donors or their relatives. Researchers are encouraged to require confidentiality when sharing genomic data that has the potential to connect donors and family members with de-identified cells and tissues.
2.3.3 Payments to Individuals Donating Cells and Tissue for Research
Recommendation 2.3.3.1: Research oversight committees must authorize all proposals to reimburse for out-of-pocket expenses to donors of embryos, sperm, or somatic cells.
Individuals who choose to provide previously stored cells and tissues for research should not be reimbursed for the costs of storage prior to the decision to participate in research. For the provision of fresh somatic cells or sperm for research, reimbursement for out-of-pocket expenses incurred by donors may be determined during the review process. For the provision of embryos or fetal tissue for research, no payment or valuable consideration of any kind beyond reimbursement of out-of-pocket expenses may be offered to donors for their procurement.
Recommendation 2.3.3.2: For the provision of oocytes for research, when oocytes are collected outside the course of clinical treatment, compensation for non-financial burdens should not constitute an undue inducement.
Because women carry particular burdens during the procurement of their gametes, their efforts should be acknowledged fairly and appropriately. At the same time, precaution is needed to avoid the potential for exploitation.
In jurisdictions where the provision of oocytes for research is legally permissible, the human subjects review committee and those responsible for conducting specialized research oversight must assess the safety and the voluntary and informed choice of women to provide oocytes for research according to the following standards:
There should be monitoring of recruitment practices of oocyte donors to ensure that the decision of women to donate their oocytes is free of undue inducement and exploitation.
In jurisdictions where research subjects are allowed compensation or valuable consideration for incurred non-financial burdens, the amount of financial recognition for the subject’s time, effort, and inconvenience must be reviewed to ensure that such compensation does not constitute an undue inducement.
Compensation for oocyte providers’ time, effort, and inconvenience, if permitted by local laws and human subjects review committees, should be reasonably consistent with compensation levels for other types of research participation involving similarly invasive and burdensome medical procedures. Compensation levels should aim to acknowledge oocyte providers’ non-financial burdens incurred as a result of their research participation, such as their physical discomfort and effort.
At no time should payments or other rewards of any kind be given for the number or quality of the oocytes that are to be provided for research.
Oocyte procurement must be performed only by medically qualified and experienced clinicians, and frequent monitoring and dose adjustment must be used to reduce the risk of ovarian hyperstimulation syndrome.
Due to the potential long-term effects of ovulation induction, women should undergo a limited number of hormonally induced ovarian stimulation cycles in a lifetime, regardless of whether they are induced for research or assisted reproduction. The limits should be determined by a thoughtful research review and oversight process, which should be informed by the latest available scientific information about the health risks.
A fertility clinic or other third party responsible for obtaining consent or collecting cells or tissues should not be paid for the material obtained. It should be eligible for specifically defined cost-based reimbursements and payments for professional services. Fertility clinics should not profit from providing tissues for research.
To help guide review committees through the ethical considerations surrounding oocyte collection and financial recognition of providers’ efforts, the ISSCR Ethics and Public Policy Committee developed an advisory report outlining their deliberations on these issues (Haimes et al., 2013).
-
Recommendation 2.4.1: Proposals for derivations of new hESC lines should be scientifically justified and executed by scientists with appropriate expertise. A clear, detailed outline for banking new lines should be incorporated into derivation proposals. Whenever feasible, the distribution of new hESC lines to the research community is strongly encouraged following derivation and first publication.
Consistent with the policies of many funders and scientific journals, researchers should deposit lines into centralized repositories where the lines will be held for release and distribution upon publication. Researchers performing derivations should have a detailed, documented plan for characterization, storage, banking, and distribution of new lines. Researchers performing derivations should propose a plan to safeguard the privacy of donors and inform donors that, in this era of data-intensive research, complete privacy protection might be difficult or impossible to guarantee.
Although a specialized oversight process is not required for derivation of non-embryonic stem cell lines, the general principles and aspirational goals for banking and distribution apply widely to all classes of scientifically valuable stem cell lines. It is understood that cell lines (e.g. pluripotent stem cells, neural stem cells, hematopoietic stem cells) made for commercial purposes may not be available for general distribution. In addition, cell lines made for autologous applications may not be suitable or available for general distribution.
Repositories & Registries for Stem Cell Lines
Recommendation 2.4.2: National and international repositories should accept deposits of newly derived stem cell lines to preserve them, maintain them to a high standard, and ensure their authenticity. Repositories are encouraged to distribute them internationally to enable their dissemination. Researchers are encouraged to deposit data on stem cell lines into registries.
Repositories should strive to adhere to common methods and standards to facilitate the easy exchange and dissemination of stem cell lines (see also Section 5, Standards in Stem Cell Research). At a minimum, each repository must establish its own guidelines and must have a clear, easily accessible material transfer agreement. A sample material transfer agreement is available in Appendix 4. Each repository may have its own criteria for distribution. Repositories should also have clear, publicly available protocols for the deposit, storage, and distribution of pluripotent stem cell lines and related materials. The repository has the right of refusal if a cell line does not meet its standards.
Repositories must require written assurances from depositors that research materials were procured according to the ethical principles and compliant with the regulations and policies of the respective jurisdiction. Depositors should attest that they were subject to appropriate oversight (IRB or equivalent) for all human subjects work, obtained informed consent from research material donors, and maintain consent documentation for the distribution and use of the research material. Provider/depositors must also provide written assurances that the MTA provided for the transfer of materials contains all restrictions, regulations, and obligations consistent with the informed donor consent for the use of the materials. Repositories must receive and preserve the material transfer agreements for any material deposit and ensure its full execution prior to the transfer of materials to the requesting investigators.
Repositories should obtain all available technical information from the depositor, for example, methods used in the derivation of lines, culture conditions, infectious disease testing, passage number and characterization data. Repositories should make this information available to researchers. If the repository modifies the depositor’s protocols or obtains additional data, this information should also be made available.
Repositories should engage in, but are not limited to, the following:
Reviewing and accepting deposit applications.
Assigning unique identifiers (catalogue number) to deposits.
Expansion, maintenance and storage of cell lines.
Quality assurance and quality control of all procedures.
Maintenance of website with pertinent characterization data, protocols and availability of cell lines.
Maintaining databases to allow tracking and distribution of the cell lines to primary researchers.
Posting a clear and fair cost schedule for distribution of materials. Repositories should endeavor to distribute internationally and charge only the necessary costs, which include shipping and handling.
Preserve cell lines for future use.
Provenance of Stem Cell Lines
Recommendation 2.4.3: Documentation of the provenance of stem cell lines is critical if the cell lines are to be widely employed in the research community. Provenance must be easily verifiable by access to relevant material transfer agreements and data demonstrating the identity of the cell line and uses allowed under the original informed consent. If a cell line has the potential to be used clinically, researchers are encouraged to provide information on the materials used for derivation and expansion.
Appropriate safeguards should be used to protect the privacy of donors and donor information due to the nature of the materials involved in the generation of human stem cell lines. For stem cell lines to be as useful as possible and so as not to preclude future potential therapeutic applications, as much donor information as possible should be maintained along with the cell line (see Recommendation 3.2.1.2). Subject to local laws, donor samples and cell lines should be anonymized or de-identified as stipulated in the informed consent forms.
Material transfer agreements or their equivalents must be consistent with the informed consent, and include all limitations, restrictions and obligations set forth by the material provider. These MTAs must be presented to the repository previous to or at the time of the material deposit and must be maintained by the repository while it holds the materials. Material providers must maintain documentation regarding consent and inform the receiving parties about relevant regulations thereof, including whatever reimbursement of direct expenses or financial or valuable considerations of any kind were provided in the course of the procurement.
Access to Research Materials
Recommendation 2.4.4: Institutions engaged in human stem cell research performed with public funding are encouraged to develop procedures whereby researchers are granted access to research materials for scientifically and ethically appropriate purposes, as determined under these guidelines and applicable laws.
Researchers are encouraged to make the materials readily accessible to the biomedical research community for non-commercial research. When arranging for the disposition of material rights generated with public funding to commercial entities, institutions are encouraged to preserve non-exclusive access for the research community. If materials are made available as a researcher-to-researcher transfer from a repository or bank, the costs of cell line expansion, handling, and shipping should be borne by the receiving party so as not to pose an undue financial burden on the entity or researcher providing the cells.
-
Recommendation 2.5.1: These guidelines should be upheld and enforced through standards of academic, professional, and institutional self-regulation.
These guidelines were developed collaboratively to form an international consensus for the ethical standards and practices in human embryo and stem cell research. These standards and practices represent a comprehensive code of conduct applicable to all researchers in the field. They are a critical catalyst that ensures international collaboration and research can proceed with confidence from anywhere in the world in a manner accepted as valid by the scientific and ethics communities.
Grant applicants, in particular the individual scientists undertaking the research, should provide funding bodies with sufficient documentation to demonstrate that the proposed research complies with relevant local and national regulations and these guidelines or their equivalent. Funding organizations should pledge to follow these guidelines or their equivalent and require entities whose research is funded by such organizations to do the same.
Senior or corresponding authors of scientific publications should specifically be charged with the responsibility of ensuring that the code of conduct embodied in these guidelines is adhered to in the course of conducting human embryo and stem cell research; this includes the supervision of junior investigators that work for their respective organizations or on their research projects. Institutions where human embryo and stem cell research is undertaken should strive to provide researchers working on any such projects under their auspices, particularly junior investigators, with current information on such standards and practices.
Ensuring that research is performed according to scrupulous ethical standards is a legitimate concern for the peer review and editorial process of scientific publication. Journal editors and manuscript reviewers may request access to research protocols and provenance documents to enable adequate review of the ethical framework and oversight of the research process and may request an authors’ statement of adherence to these or an equivalent set of guidelines or applicable regulations. Authors should include a statement that the research was performed after obtaining approvals following a suitable research oversight process.
Finally, as stated previously, the ISSCR has made available for download examples of informed consent documents for obtaining human materials for research (gametes, embryos, and somatic cells) and a material transfer agreement for the sharing and distribution of materials in order to facilitate the adoption of globally accepted standards and practice of human embryo and stem cell research (Appendices 2 and 3). These templates may be modified to comply with the policies and regulations in their respective jurisdiction.
Return to TOC | 2021 Guidelines for Stem Cell Research and Clinical Translation
3. Clinical Translation of Stem Cell-based Interventions
Stem cell and embryo research show great promise for advancing our understanding of human development and disease, including research to address issues pertinent to the earliest stages of human development
Return to TOC | 2021 Guidelines for Stem Cell Research and Clinical Translation
This section highlights the scientific, clinical, regulatory, ethical, and social issues that should be addressed so that basic stem cell research is responsibly translated into appropriate clinical applications.
The rapid advances in stem cell research and genome editing technologies have created high expectations for the promise of regenerative medicine and gene- and cell-based therapies. As the field advances, it is important to balance the excitement from patients, scientists, clinicians, and the media over the growing number of clinical trials for serious diseases with the requirement to rigorously evaluate the safety and effectiveness of each potential new intervention. There have been instances where some clinical applications and clinical trials occurred far in advance of what is warranted by sound, rigorous, and dispassionately assessed preclinical evidence. Clinical experimentation is burdensome for research subjects and expensive; therefore, new interventions should only advance to clinical trials when there is a compelling scientific rationale, a plausible mechanism of action, and an acceptable chance of success. Furthermore, the safety and effectiveness of new interventions must be demonstrated in well-designed and expertly-conducted clinical trials with approval by regulators before the interventions are offered in direct-to-consumer settings to patients or incorporated into standard clinical care. Finally, any premature clinical testing of a promising new technology may jeopardize its further development if some adverse event emerges due to inadequate trial design or product manufacturing. Stem cell science is best positioned to fulfill its potential by adhering to a commonly accepted and robust set of guidelines for evidence-based therapy development.
-
Recommendation 3.1.1: Stem cells, cells, and tissues that are substantially manipulated or used in a non-homologous manner must be proven safe and effective for the intended use before being marketed to patients or incorporated into standard clinical care.
The therapeutic use of substantially manipulated stem cells, cells, or tissues or minimally manipulated stem cells, cells, or tissues for non-homologous treatments is complex, speculative, and has been shown to have risks to recipients. These products should be thoroughly tested in preclinical and clinical studies and evaluated by regulators as drugs, biologics, and advanced therapy medicinal products.
Minimally Manipulated Stem Cells, Cells, and Tissues
Minimally manipulated cells and tissues, such as, in some cases, fat tissue transferred from one part of the body to another, are generally subject to fewer regulatory requirements. When a stem cell-, cell-, or tissue-based intervention is claimed to be minimally manipulated and exempt from regulatory oversight on this basis, the responsibility rests on the clinician to invite independent scrutiny of their process of manipulation, such that scientific and regulatory experts can determine the proper level of regulatory oversight. When there is uncertainty or disagreement about the regulatory status of particular interventions, it is best to contact legally authorized regulatory bodies and seek their guidance concerning how specific interventions are classified. The US Food and Drug Administration, European Medicines Agency, Australian Therapeutic Goods Administration, Japanese Ministry of Health, Labour, and Welfare, and other regulators have released detailed standards to delineate when manipulation of cell-based products can no longer be considered minimal or their use homologous, and must therefore be subject to regulatory oversight as an advanced therapy product.
Substantially Manipulated Stem Cells, Cells, and Tissues
Substantially manipulated stem cells, cells, and tissues are subjected to processing steps that alter their original structural or biological characteristics. These processes can include isolation and purification processes, tissue culture and expansion of the cells, genetic manipulation, or other steps. For example, the extraction of cells from adipose tissue using enzymatic digestion, ultrasonic cavitation, or other means involves processing steps that can alter the original function of the cells imbedded in the tissue. The safety and efficacy profile of such an intervention needs to be determined for its particular indication using rigorous research methods. Safety and efficacy cannot be assumed because the composition of the intervention may differ from the original source tissue. Demonstration of safety and effectiveness will depend on the particular intervention and the specific condition targeted. Both to protect patients from risks and to help ensure that promising interventions are studied, it is critical that cells and tissues that have been substantially manipulated are evaluated by national regulators as drugs, biologics, and advanced therapy medicinal products.
Non-homologous Use of Stem Cells, Cells, and Tissues
Non-homologous use occurs when the stem cells, cells, or tissue are repurposed to perform a different basic function in the recipient than the cells or tissue originally performed prior to being removed, processed, and transplanted or otherwise delivered. For example, delivering adipose-derived stromal cells into the eye with the intent to treat macular degeneration would be a non-homologous use because the basic function of adipose tissue is not the trophic support of the retina. As with substantially manipulated cells and tissues, the non-homologous use of stem cells, cells, and tissues has potential benefits but can also pose serious risks. In the case of using adipose-derived stromal cells to treat macular degeneration, for example, there are well-documented reports of vision loss (Kuriyan et al., 2017). Such reports serve as a reminder that cells and tissues, depending on how they are administered, can cause serious harm. The benefit-risk ratio for non-homologous uses will depend on the particular intervention and the specific use. To protect patients from risks, and to ensure that necessary research is conducted, it is important that the safety and effectiveness of non-homologous uses be rigorously evaluated by regulators following the completion of well-designed and carefully controlled preclinical and clinical studies.
-
In most jurisdictions, the use of cellular products for medical therapy is regulated by governmental agencies to ensure the protection of patients. Although some stem cell-based products have now been approved for use in humans, a growing number of novel cellular products are being tested for a wide range of disease indications and present new challenges in their processing, manufacture, and pathways for regulatory approval. Given the variety of potential stem cell-based interventions, these guidelines emphasize that cell processing and manufacture of any product be conducted with scrupulous, expert, and independent review and oversight, to ensure the integrity, function, and safety of cells destined for use in patients. Manufacture of cells outside the human body introduces an additional risk of contamination with pathogens, and prolonged passage in cell culture carries the potential for accumulating mutations and genomic and epigenetic instabilities that could lead to altered cell function or malignancy, especially as such cells may outgrow others in the cultures. While many countries have established regulations that govern the culture, genetic alteration, and transfer of cells into patients, optimized standard operating procedures for cell processing, protocols for characterization, and criteria for release remain to be refined for the products of emerging technologies such as genome editing and novel derivatives of pluripotent cells and many attendant cell therapies.
Given the unique proliferative and regenerative nature of stem cells and their progeny and the uncertainties inherent in the use of this therapeutic modality, stem cell-based therapies present regulatory authorities with unique challenges that may not have been anticipated within existing regulations. The following recommendations involve general considerations for cell processing and manufacture.
3.2.1 Sourcing Material
Donor Consent
Recommendation 3.2.1.1: Donors of cells for allogeneic use should give written and legally valid informed consent that covers, where applicable, terms for potential research and therapeutic uses, disclosure of incidental findings, potential for commercial application, and issues specific to the type of intervention under development.
Researchers should ensure that potential donors or their legally authorized representatives adequately understand the stem cell-specific aspects of their research participation. For a list of donor informed consent discussion points, see Section 2.3.2 and Appendix 3.
The initial procurement of tissue from a human donor may or may not require good manufacturing practice (GMP) certification depending on the jurisdiction, but this should always follow regulatory guidelines related to human tissue procurement and maintain universal precautions to minimize the risks of contamination, infection, and pathogen transmission.
Donor Screening
Recommendation 3.2.1.2: Donors and/or the resulting cell banks developed for allogeneic stem cell-based interventions should be screened and/or tested as applicable for infectious diseases and other risk factors, in compliance with applicable regulatory guidelines (see Recommendation 2.4.3).
Tissue procurement for generating stem cell-based interventions is similar to procurement of cells/tissues for other potential clinical purposes and should be governed by the same policies. However, an important distinction between tissue donation and stem cell generation that increases the importance of screening is that, while tissues and organs other than blood are usually distributed to a limited number of recipients, somatic or pluripotent cells derived from allogeneic cells or tissues can potentially be implanted into a large number of patients. Donor screening should include medical examination, collection of donor history and blood testing. This process mitigates the risk of potential transmission of adventitious agents from the donor to patients receiving the stem cell products. Regulatory agencies such as the (FDA; https://www.fda.gov/) and the European Medicines Agency (EMA; https://www.ema.europa.eu/) have issued guidance regarding donor testing and screening. If high-specificity tests are available for adventitious agents, direct testing of the donated cells and tissue can mitigate the need to screen for such agents. However, this type of testing strategy should be prospectively discussed with regulatory authorities to ensure appropriate risk mitigation.
In some cases, in may not be possible to screen donors. For example, the donation of human embryos for the derivation of hESCs often occurs years after the harvesting of gametes and generation of the embryos, consistent with ethical and regulatory standards. Therefore, screening of the donors at the time of gamete harvest is not appropriate. In these cases, the hESC cell bank can be thoroughly tested to ensure the absence of adventitious agents. However, there still remains the risk of pathogens for which validated tests are not available.
3.2.2 Manufacture
Cellular derivatives generated from stem cells and tissues are considered manufactured products and are subject to various regulations to ensure their quality (consistency, purity, and potency) and safety.
Quality Control in Manufacture
Recommendation 3.2.2.1: All reagents and processes should be subject to quality control systems and standard operating procedures to ensure the quality of the reagents and consistency of protocols used in manufacturing. Manufacturing should be performed under GMP conditions when possible or mandated by regulation. However, in early-stage clinical trials it is understood that GMPs may be introduced in a phase appropriate manner in some regions.
The variety of distinct cell types, tissue sources, and modes of manufacture and use necessitate individualized approaches to cell processing and manufacture. The maintenance of cells in culture for any period of time places selective pressures on the cells that are different from those in vivo. Cells in culture age and may accumulate both genetic and epigenetic changes, as well as changes in differentiation behavior and function. Scientific understanding of genomic stability during cell culture and assays of genetic and epigenetic status of cultured cells are still evolving. Guidance documents from the FDA and EMA, as well as other documents, provide a roadmap for manufacture and quality control of cellular products. However, given that many cellular products developed in the future will represent entirely novel entities with difficult-to-predict behaviors, scientists must work with regulators to ensure that the latest information is available to inform the regulatory process. An important goal is the development of universal standards to enable comparisons of cellular identity, purity and potency, which are critical for comparing studies and ensuring reliability of dose-response relationships and assessments of mechanisms of toxicity.
Processing and Manufacture Oversight
Recommendation 3.2.2.2: The oversight and review of cell processing and manufacturing protocols should be rigorous, and consider the manipulation of the cells, their source and intended use, the nature of the clinical trial, and the research subjects who will be exposed to them.
Stem cells can proliferate in culture for extended periods of time. This proliferative capacity carries risks. When maintained in culture for prolonged periods of time, cells may acquire mutations, grow and differentiate into inappropriate cellular phenotypes, form benign or malignant outgrowths, and fail to mature. Appropriate tests must be devised to maximize safety of stem cell derived products.
Factors that are common to many stem cell products include the cells’ proliferation and differentiation potentials, source (autologous, allogeneic), type of genetic manipulation, if any, homologous versus non-homologous or ectopic use, their persistence in the recipient, and the anticipated integration of cells into tissues or organs (versus, for example, encapsulation). Culture composition and purity of desired phenotype vs. extent of residual undifferentiated progenitors should be carefully evaluated. In order to mitigate the risk associated with these factors, stem cell-based interventions should be thoroughly tested in safety and efficacy preclinical studies. Given that each stem cell therapy is a unique product, the evaluation of each product should be informed by the characteristics of the cell product and the risk/benefit associated with the clinical indication.
Assays available for genetic and epigenetic assessment of stem cell-based products are evolving. Researchers should be aware of the limitations of these assays in predicting clinical outcomes. For cryopreserved or otherwise stored products, any impact of short- or long-term storage on product potency and stability must be determined. Human or xenogeneic materials associated with elevated risk (for example, human allogeneic and pooled source materials or xenogeneic reagents such as fetal bovine serum) should be stringently tested for safety and quality.
Components in Culture or Preservation of Cells
Recommendation 3.2.2.3: Human or chemically defined components should be used in the culture or preservation of cells whenever possible.
Cells are likely to be expanded in culture and might be exposed to xenogeneic materials before transplantation. Components of non-human animal origin present risks of transferring pathogens or unwanted biological material and can be quite variable in composition and bioactivity. As such the risk of transmission of viruses and other infectious agents is proportionately greater when using xenogeneic materials. Researchers can mitigate this risk by properly sourcing xenogeneic reagents from regions reasonably assumed to be clear of known pathogens. If xenogeneic components cannot be reasonably removed, researchers should demonstrate the lack of feasible alternatives and document favorable risk/benefit in using animal-based components. These risks can be mitigated by using reagents in which the manufacturers have included pathogen reduction steps which remove pathogens (such as viral inactivation), or testing cell lines (such as CHO lines), used in the manufacture of these reagents. In addition, adventitious agent testing of the cells should include testing for appropriate xenogeneic pathogens; these requirements are specified in guidance documents published by the FDA, EMA and other regulatory agencies. Careful adherence to regulations and tracking of cells and reagents and the development of a risk mitigation plan is crucial to translation and uptake of cell-based therapies.
Recommendation 3.2.2.4: All reagents used in manufacturing stem cell-derived therapeutics should be of the highest quality available.
In order to ensure the safety of stem cell products, the reagents and raw materials used in manufacturing should be manufactured under GMPs, whenever possible. It should be noted that while manufacturing under GMPs ensures product consistency and purity, it does not necessarily assure absence of adventitious agents. Therefore, a risk mitigation assessment and adventitious agent testing plan should be performed that address risks associated with all reagents used in manufacturing.
In some cases, GMP grade reagents may not be available. In these cases, it is recommended to use reagents that comply with compendial requirements (e.g., USP, British Pharmacopoeia) and which were manufactured using the highest level of controls possible. It may also be necessary to clarify the appropriateness of reagents and raw materials with regulatory agencies if there are doubts about whether reagents that are not made under GMPs are of a sufficiently high enough quality for human use. It is essential that documentation including lot numbers and certificates of analysis and certificates of origin be retained for every reagent used in the isolation, expansion, and manipulation of stem cells that will eventually be used in the generation of therapeutic products.
Release Criteria
Recommendation 3.2.2.5: Criteria for in process and release specifications should be developed during the regulatory review process. Culture-acquired genetic abnormalities may be a significant risk and should be part of in process and/or final product testing for stem cell products that have undergone extensive expansion in vitro.
The genetic and epigenetic stability of pluripotent stem cell-derived products warrants careful scrutiny. During manufacturing, it will be important to test cytogenetic abnormalities, as well as additional genetic and epigenetic parameters as defined by the protocol review process. The limitations of any such tests will be assessed and weighed against the risk /benefit and patient population for any given case.
Recommendation 3.2.2.6: Criteria for release of cells should include the assessment of off-target cells, using the most sensitive assays possible.
Release criteria for stem cell-based interventions should utilize qualified or validated assays that assess the identity, purity, sterility, activity, and potency of the product. Because stem cell products may consist of heterogeneous populations of cells, it is important to include assays that detect and quantify the target cells responsible for the bioactivity of the product as well as other “off-target” cell populations.
The off-target cells may be cells from different lineages, cells from the same lineage, partially differentiated cells, or undesirable cells such as undifferentiated stem cells. For pluripotent stem cell-derived products, there is concern about residual undifferentiated pluripotent stem cells that may remain in the product. Given the nature of pluripotent stem cells and their innate capacity to form teratomas, there is a particular concern for the potential tumorigenicity of stem cell-based interventions. Therefore, the development of sensitive assays to detect contaminating undifferentiated pluripotent stem cells in the final product is critical, especially when delivering large cell doses. Further, the sensitivity of these assays should be documented in regulatory submissions. Some techniques (such as FACS analysis) may be suitable for doses of millions of cells but for doses in the 108-109 range more sensitive assays may need to be developed.
In summary, all stem cell-based interventions should be defined with their constituents as completely as possible, including at a minimum the proportion of therapeutic (on target) cells within the final cell product as well as minimizing the cells capable of causing major side effects, including tumor formation.
-
The purpose of preclinical studies is to (a) provide evidence of product safety and (b) establish proof-of-principle for therapeutic effects. International research ethics policies, such as the Declaration of Helsinki (1964) and the Nuremberg Code (1949), strongly encourage the performance of non-human animal studies prior to clinical trials. Before initiating clinical studies with stem cell-based interventions in humans, researchers should have persuasive evidence of safety and the potential for clinical utility in appropriate in vitro and/or animal models. These preclinical studies must be rigorously designed and have been subject to regulatory oversight and reviewed independently prior to the initiation of clinical trials. This helps ensure that trials are scientifically, medically, and ethically warranted.
Cell-based interventions offer unique challenges for preclinical studies. In some cases, homologous cells in the same species are unavailable. Immune-suppressed animal models, while useful, do not permit an understanding of the effect of the immune system on transplanted cells, or, more often, they may not share all the same biological properties of their human counterparts. Since transplanted cells are considerably more complex and can change after transplantation in unpredictable ways, extrapolating cell therapies in an animal model to humans is even more challenging than for small molecule therapeutic candidates.
-
Animal Welfare
Recommendation 3.3.1.1: Preclinical research into stem cell-based interventions involving animals should adhere to the principles of the three Rs: reduce numbers, refine protocols, and replace animals with in vitro or non-animal experimental platforms whenever possible.
This recommendation is not incompatible with performing replication experiments or achieving adequate statistical power (see: www.nc3rs.org.uk). Indeed, these are key steps for ensuring that animal experiments support robust conclusions. This recommendation should also not be interpreted as suggesting that in vitro or non-animal platforms are sufficient for supporting clinical investigations. For most stem cell therapies safety testing should be performed in vivo. However, recent developments in organoid systems suggest that efficacy testing in vitro may by suitable in some circumstances.
Preclinical Study Objectives
Recommendation 3.3.1.2: Early phase human studies should be preceded by a rigorous demonstration of safety and efficacy in preclinical studies. These preclinical studies can include in vitro and in vivo modeling.
Preclinical efficacy studies help provide the scientific rationale for proceeding into human trials. Stringent design and reporting standards should be demanded where planned trials involve invasive delivery approaches or where the cell product presents greater risk and uncertainty. However, prudent use of scientific resources means that even when risk is modest, studies should rest on sound scientific evidence of expected efficacy.
The development of stem cell-based products generally includes a period of process development in which the manufacturing process is optimized. This may include exchanging research grade reagents with reagents manufactured under GMP and removing xenogeneic reagents from the process. Because these changes can affect the cellular composition and bioactivity of the final product, it is important that preclinical efficacy studies use stem cell product manufactured using processes intended for clinical applications, whenever feasible.
Study Validity
Recommendation 3.3.1.3: All preclinical studies testing safety and efficacy should be designed in ways that support precise, accurate, and unbiased measures of potential clinical utility. In particular, studies designed to inform trial initiation should have high internal validity; they should be as representative as possible of clinical scenarios they are intended to model, and they should be replicated.
Preclinical experiments confront many sources of bias and confounding factors, including selection bias. For decades, clinical researchers have sought to minimize the effects of bias and confounding by using techniques like randomized allocation, blinded outcome assessment, or power calculations. Such rigor should also apply in preclinical studies intended to support trials. Numerous groups have articulated standards for designing preclinical studies aimed at supporting trials (Fisher et al., 2009; Henderson et al., 2013; Landis et al., 2012; Kimmelman et al., 2014). Key design principles include:
Researchers should reduce bias and random variation by ensuring their studies have adequate statistical power, use appropriate controls, randomization, and blinding, and, where appropriate, establish a dose-response relationship.
Critical or definitive safety and efficacy studies should be performed with prospective protocols and should have independent quality oversight.
Researchers and sponsors should ensure preclinical studies model clinical trial
settings. Researchers should characterize disease phenotype at baseline, select animal models that best match the human disease, use outcome measures that best match clinical outcomes, and provide evidence supporting a mechanism for treatment effect.
Researchers and sponsors should ensure effects in animals are robust by replicating findings, ideally in an independent laboratory setting.
Researchers and sponsors should pre-specify and report whether a study is exploratory (i.e., hypothesis generating or aimed at substantiating basic science claims) or confirmatory (i.e., using pre-specified hypotheses and protocols and powered to support robust claims). Preclinical researchers should only venture claims of potential clinical utility after confirmatory studies.
Sex as a Biological Variable
Recommendation 3.3.1.4: Preclinical studies should assess both male and female animals in safety and efficacy testing unless there is a scientifically valid reason not to do so.
Males and females can respond differently to medical treatments as well as to the incidence of diseases, which can reflect distinct underlying pathways and mechanisms, due to chromosomal makeup and effects of gonadal hormones. Therefore, it is important to include both male and female animals in preclinical efficacy and safety studies. Of particular importance is the inclusion of both sexes in long-term safety studies, which is typically a mandatory requirement from many funding and regulatory agencies. In vitro model systems should, whenever possible, also be derived from male and female cells.
-
Human cells should be produced under the conditions discussed in Section 3.2, Cell Processing and Manufacture. Depending on the laws and regulations of the specific region, biodistribution and toxicity studies should be performed using good laboratory practice (GLPs). It is recommended that these studies be performed by a third party, such as a Contract Research Organization (CRO).
Cell Characterization
Recommendation 3.3.2.1: Cells to be employed in clinical trials must first be rigorously characterized to assess potential toxicities through studies in vitro and, where possible, for the clinical condition and tissue physiology to be examined in animal models.
Outside of the hematopoietic, stratified epithelia, and various stromal cell systems there is little clinical experience with the toxicities associated with infusion or transplantation of stem cells or their derivatives. In addition to known and anticipated risks (for example, acute infusional toxicity, immune reactions, and tumor development), stem cell-based interventions present risks that will only be discovered with experience. As non-human animal models may not replicate the full range of human toxicities associated with stem cell-based interventions, particular care must be applied in preclinical analysis. This section defines toxicities that are likely to be unique to stem cells or their progeny.
Tumorigenicity Studies
Recommendation 3.3.2.2: Risks for tumorigenicity must be rigorously assessed for any stem cell-based product, especially if cells are extensively manipulated in culture, genetically modified, or when derived from a pluripotent source.
Assessing tumorigenicity is a critical part of determining the safety profile of stem cell products. These studies can be challenging, as they usually require assessment of the human cell product in xenogeneic models. Further, these studies usually include long-term time points that can be several months to years. Therefore, immunocompromised animals, usually rodents, are often the animal model of choice.
All stem cell derived products should be tested for tumorigenicity, see Recommendation 3.2.2.5. Long-term animal studies are necessary to demonstrate that the persistence of any remaining undifferentiated cells in the final product do not result in tumors.
It is understood that assessing tumorigenicity in animal models is complicated by implantation technique, composition of the test article (percentage of undifferentiated cells versus the percentage of cell product), and various other parameters. Because of this complexity, tumorigenicity studies may benefit from additional in vitro studies. These would include examining rates of proliferation, observing if faster dividing subclones tend to take over the cultures, and looking for expression of oncogenes or loss of tumor suppressor gene activity. However, while these tests may add to in vivo studies, they cannot substitute for them.
Positive tumor-generating controls and negative controls assessing background tumorigenesis should be run in parallel in these studies to validate results. Specifically, this informs whether the site of implantation and other delivery parameters are permissive to tumor formation, allowing interpretation of a negative result. In these studies, it is important to deliver the cell product to the intended clinical site, if feasible. Further, assessment of the clinical dose is also important. In cases where the human dose includes very large quantities of cells, this can be quite challenging, and it is critical to work with regulators to ensure that proposed study designs are appropriate. For example, in cases where it is not feasible to deliver a human-sized dose into an immunocompromised animal model, the risk from residual undifferentiated cells in the product may be assessed by spiking the largest feasible animal dose of the therapeutic product with the highest number of undifferentiated cells that might be present in the human-sized dose (based on the sensitivity of the assay used for measuring their presence in the clinical dose).
The plan for assessing risks of tumorigenicity should be reviewed and approved by regulators before initiation of definitive preclinical studies and clinical trials. For additional guidance on specific techniques that may be of utility for genome edited interventions, see Appendix 5.
Biodistribution Studies
Recommendation 3.3.2.3: For all stem cell-based products, whether injected locally or systemically, researchers should perform detailed and sensitive biodistribution studies of cells.
Because of the potential for cells to persist or expand in the body, investigators must seek to understand the nature and extent by which cells distribute throughout the body, lodge in tissues, expand and differentiate. Careful studies of biodistribution, assisted by ever more sensitive techniques for imaging and monitoring of homing, retention and subsequent migration of transplanted cell populations is imperative for interpreting both efficacy and adverse events. These studies should whenever feasible, include delivery of the cell product using the intended clinical route and site of delivery.
Additional histological analyses or banking of organs for such analysis at late time points is recommended. Depending on the laws and regulations within specific jurisdictions, biodistribution and toxicity studies may need to be performed in a good laboratory practice (GLP)-certified animal facility.
Distinct routes of cell administration, local or systemic, homologous or non-homologous/ectopic, can lead to different adverse events. For example, local transplantation into organs like the heart or the brain may lead to life-threatening adverse events related to the transplantation itself or to the damage that transplanted cells may cause to vital structures. Especially in cases where cell preparations are infused at anatomic sites distinct from the tissue of origin (for example, for non-homologous use), care must be exercised in assessing the possibility of local, anatomically specific and systemic toxicities.
Ancillary Therapeutic Components
Recommendation 3.3.2.4: Before launching high-risk trials or studies with many components, researchers should establish the safety and optimality of other intervention components, like devices or co-interventions such as surgeries.
Cell-based interventions may involve other materials besides cells, such as biomaterials, engineered scaffolds, and devices. There may also be co-interventions like surgery, tissue procurement procedures, and immunosuppression. Additional components added to the cellular product, or delivery device can interact with the stem cell product and each other. In these cases, safety and efficacy studies should include the assessment of the final combination product. Many subjects in cell-based intervention studies may be receiving immunosuppressants or drugs for managing their disease. These can also interact with the implanted cell product. Safety and efficacy studies should include assessment of possible interactions between the cell product and these types of medications, in vitro or in vivo.
Long-term Safety Studies
Recommendation 3.3.2.5: Researchers should adopt practices to address long-term risks in preclinical studies.
Given the likelihood for long-term persistence of cells and the irreversibility of some cell-based interventions, testing of the long-term effect of cell transplants in animal models is encouraged.
Application of genetic alteration and genome editing technologies to stem cell products
Recommendation 3.3.2.6: Researchers should comprehensively investigate the type, extent and genomic distribution of introduced genetic alterations as well as their potential adverse effects on the genome and the biological properties of the treated cells at short and long-term time points.
Genetic alteration and genome editing technologies can be coupled to stem cell therapies or applied directly in vivo to resident tissue cells for a variety of therapeutic purposes.
Gene replacement approaches have made substantial progress and advances into clinical testing, either performed ex vivo on hematopoietic stem cells, lymphocytes or epidermal stem cells or in vivo targeting the liver, retina or CNS, with a growing number of therapies approved for market access. Targeted genome editing strategies are still in the early stage of clinical development, although there is constant progress and early clinical testing is showing safety and some efficacy, at least for ex vivo based strategies.
Considering the likelihood for long-term persistence, expansion and broad clonogenic output of many stem cell types and the irreversibility of any genetic alteration introduced by integrating gene transfer or genome editing, the type, extent and genomic distribution of the introduced genetic alteration, including on- and off-target events, should be comprehensively investigated as well as their potential adverse effects on the genome and the biological properties of the treated cells both in the short- and long-term. This is particularly important following genome editing when this has involved double strand breaks in DNA; the analysis of manipulated cells should include an assessment of incorrect on- and off-target events, and whether these pose any risk. Whenever possible and scientifically appropriate, such testing should include cell transplants in suitable xenogeneic hosts for long-term observation.
Potential of Stem Cells for Toxicology
Recommendation 3.3.2.7: Researchers, sponsors, and regulators should take advantage of the potential for using stem cell-based systems to enhance the predictive value of preclinical toxicology studies.
Stem cell science offers the prospect of testing toxicology in cell-based systems or artificial organs that more faithfully mimic human physiology than animal models. Such approaches, though unlikely to ever completely substitute for in vivo testing in animals, hold substantial promise for reducing burdens imposed on animals in safety testing and improving the predictive value of preclinical safety studies.
-
Given the therapeutic goals of stem cell-based interventions, preclinical studies should demonstrate evidence of therapeutic effect in a relevant animal model for the clinical condition and the tissue physiology to be studied. Mechanistic studies utilizing cells isolated and/or cultured from animal models or human tissues, both diseased and controls, are critical for defining the underlying biology of the cell-based interventions. However, a complete understanding of the biological mechanisms at work after stem cell-based intervention is not a prerequisite to initiating trials, especially when trials involve serious and untreatable diseases for which efficacy and safety have been demonstrated in relevant animal models and/or in conclusive human studies with the same cell source. Further, in rare cases, appropriate animal models may not exist. In these cases, in vitro studies may be used to support the rationale for potential efficacy.
Efficacy Evidence for Initiating Trials
Recommendation 3.3.3.1: Trials should generally be preceded by compelling preclinical evidence of clinical utility in well-designed studies. Animal models suited to the clinical condition and the tissue physiology should be used, unless there is evidence of efficacy using similar products against similar human diseases, or if it is not feasible to establish appropriate or predictive animal models.
Rigorous preclinical testing in animal models is especially important for stem cell-based approaches because cell therapies have distinctive pharmacological characteristics. Before clinical testing, preclinical evidence should ideally provide the following:
Mechanism of action. Preclinical studies should establish evidence connecting a cell-based intervention’s therapeutic activity in animal models to a pathophysiological process. These studies establish the localization of transplanted cells and provide evidence that the predicted localization is tied to the proposed mechanism of action.
Optimal conditions for applying the stem cell-based intervention (for example, dose, co-interventions, delivery).
Ability to modify disease or injury when applied in suitable animal systems, and under conditions that are similar to expected trials (see design principles under Section 3.3.1.3, Study Validity).
Sufficient magnitude and durability of disease modification or injury control to be clinically meaningful.
In cases where an intervention is substantially similar to one that has already been tested in humans, trial evidence may reduce the demand for preclinical evidence.
Animal Studies
Recommendation 3.3.3.2: Appropriate animal models should be selected which allow the assessment of efficacy and safety of the stem cell-based intervention. Safety testing should include assessment of the delivery procedure or surgical technique used for implantation of the cells.
Immune-deficient rodents or those manipulated to have humanized immune systems can be especially useful to assess human cell transplantation outcomes, engraftment in vivo, stability of differentiated cells, and cancer risk. Many small animal models of disease can faithfully reproduce aspects of human diseases, although there are considerable limitations. Small animal studies should also attempt to correlate cell number and potency required for large animal studies and subsequent trials.
Large animals may better represent human physiology as they are often genetically outbred, maintained in more diverse environments, and anatomically more similar. They may provide the opportunity to test co-interventions used in trials (for example, adjunctive immunosuppressive drug therapy) methods of introduction or the compatibility of surgical devices and cell products. They also may be essential to evaluate issues of manufacturing scale up, or anatomical factors that are likely to mediate a therapeutic effect (for example, bone, cartilage, or tendon in a load-bearing model). Trials involving risky or novel approaches should generally be supported by evidence from large animal models in cases where such models better recapitulate human disease and human anatomy (e.g., cardiomyocyte transplantation).
The need for invasive studies in non-human primates should be evaluated on a case-by-case basis, performed only if trials are expected to present high risk, and where non-human primates are expected to provide information about cell-based interventions not obtainable with other models. All studies involving the use of non-human primates must be conducted under the close supervision of qualified veterinary personnel with expertise in their care and their unique environmental needs. Particular care should be taken to minimize suffering and maximize the value of studies by using rigorous designs and reporting results in full.
-
Recommendation 3.3.4.1: Sponsors, researchers, and clinical investigators should publish preclinical studies in full and in ways that enable an independent observer to interpret the strength of the evidence supporting the conclusions.
The publication of preclinical studies serves many ends. It enables peer review of clinical research programs, thus enhancing risk/benefit ratios in trials, respects the use of animals and reagents by disseminating findings from studies, enables more sophisticated interpretation of clinical trial results, and makes possible the evaluation of preclinical models and assays, thus promoting a more effective research enterprise. However, many studies show biased patterns of preclinical publication (Sena et al., 2010; Tsilidis et al., 2013). Preclinical studies—at least those that are aimed at confirming the hypotheses motivating a development program—should be reported in full regardless of whether they confirm, disconfirm, or are inconclusive with respect to the hypothesis they are testing. These guidelines recognize that publication may reveal commercially sensitive information and therefore acknowledge that a reasonable delay is permissible to secure appropriate protection of intellectual property. Nevertheless, preclinical studies supporting a trial should be published before the first report of trials. Animal studies should be published according to well-recognized standards, such as the ARRIVE (Animal Research: Reporting In Vivo Experiments) criteria, that have been endorsed by leading biomedical journals (Percie du Sert et al., 2020).
-
The rights and welfare of human subjects participating in clinical trials must be protected in any clinical research, including trials involving stem cell-based interventions and novel reproductive technologies. Clinical research should be designed to generate scientifically rigorous information that will be used to guide important decisions for patients, clinicians, clinical investigators, sponsors, and policymakers.
Sponsors, investigators, host institutions, oversight bodies, and regulators bear responsibility for ensuring the ethical conduct of clinical trials. In addition, members of the broader research community have a responsibility for encouraging ethical research conduct. As with all clinical research, clinical trials of stem cell-based interventions must follow internationally accepted principles governing the ethical design and conduct of clinical research and the protection of human subjects (Department of Health, and Education and Welfare, 1979; European Parliament and Council of the European Union, 2001; World Medical Association, 1964; Council for International Organizations of Medical Sciences 2016). Key requirements include having adequate preclinical data, a rigorous research design that minimizes risk, independent oversight and peer review, fair subject selection, informed consent, research subject monitoring, auditing of study conduct, and trial registration and reporting. Ideally this should include the involvement of the relevant patient/carrier groups.
Some interventions and conditions present challenges for standard trial designs. Nevertheless, research in such settings should similarly involve a pre-specified protocol, independent review for scientific merit and ethics, and a plan for reporting. Translational research on novel assisted reproductive technologies ideally combines both specialized oversight process (see Section 2.1) and human subjects review.
What follows in this section pertains to clinical trials as well as innovative care pathways and observational studies.
-
The overarching goal of research oversight is to ensure that the research is safe, protects human subjects, has scientific and medical merit, and is designed and carried out to yield credible data and enhance scientific and medical understanding.
Prospective Review
Recommendation 3.4.1.1: All research involving clinical applications of stem cell-based interventions must be subject to prospective review, approval, and ongoing monitoring by independent human subjects research review committees.
Independent prospective review and monitoring are critical for ensuring the ethical basis of research with human subjects, regardless of funding source. A competent review will be predicated on the absence of conflicts of interest (both financial and non-financial) that can bias assessment of the research design, maximize the alignment of the goals of the research with the subjects’ rights and welfare, and promote valid informed consent.
Additional independent evaluation of the research may occur through other groups, including granting agencies, peer review, the specialized oversight process (see Section 2.1), regulators, and data and safety monitoring boards. Of crucial importance is that these groups collectively have the scientific, medical, and ethical expertise to conduct necessary review and oversight. To initiate stem cell-based clinical research, investigators must also follow and comply with local and national regulatory approval processes.
Expert Review of Clinical Research
Recommendation 3.4.1.2: The review process for stem cell-based clinical research should ensure that protocols are vetted by independent experts who are competent to evaluate (a) the in vitro and in vivo preclinical studies that form the basis for proceeding to a trial and (b) the design of the trial, including the adequacy of the planned endpoints of analysis, statistical considerations, and disease-specific issues related to human subjects protection.
Peer review as well as institutional review boards/research ethics committees should also judge whether the proposed stem cell-based clinical trial is likely to lead to important new knowledge or an improvement in health. Comparing the relative value of a new stem cell-based intervention to established modes of therapy is integral to the review process. Peer review should be informed, where feasible, by a systematic review of existing evidence supporting the intervention including a review of its utility against other therapies that already exist for that condition. If decisions must be made based solely on expert opinion because no relevant literature is available, this should be described explicitly in the recommendations regarding a particular trial.
-
Risk-Benefit Analysis
Recommendation 3.4.2.1: Risks should be identified and minimized, unknown risks acknowledged, and potential benefits to subjects and scientific understanding estimated. Sponsors should be able to justify research with human subjects in terms of likely risk and benefit based on evidence from preclinical studies and the published literature.
Efficient designs that minimize risks and include the minimum number of subjects required to properly answer the scientific questions at hand should be employed. Eligibility criteria in prelicensure stages should be designed to minimize risks with consideration of potential comorbidities that may increase risk or modify the risk/benefit ratio. Correlative studies should be performed to ensure that the maximum possible information is obtained on the safety and efficacy of the approach being tested, provided that such assessments do not pose an undue burden for the subject.
Systematic Appraisal of Evidence
Recommendation 3.4.2.2: Initiation of clinical trials should be supported by a systematic appraisal of evidence supporting the intervention and the current unmet need for treatment of the disease or disorder.
Decision-making about whether to proceed with a given research effort should be supported by a systematic review of available scientific evidence. At a minimum, this review should consist of a synthesis of a systematic search of published and unpublished studies testing the intervention in animal systems. For early-phase clinical trials, the systematic review will mostly involve synthesizing basic and preclinical investigations, while for late phase studies the systematic review should include clinical evidence. The systematic review should also be informed by accessing and synthesizing findings involving the testing of similar intervention strategies as well as current standard of care. Trial brochures should summarize the information gathered from systematic review without any bias.
Objectives of Trials
Recommendation 3.4.2.3: Stem cell-based interventions must be aimed toward being clinically competitive with existing therapies or meeting a unique therapeutic demand. Being clinically competitive necessitates having reasonable evidence that existing treatments are less than optimal or pose burdens that may be overcome should the stem cell-based intervention prove to be safe and effective
The rationale for developing a new stem cell-based intervention is that it can work better or as well as existing treatments with less morbidity and a favorable cost benefit analysis. Simply being able to make a therapy for a condition is not sufficient for going to a clinical trial if effective treatments already exist for patients, which have been shown to have a major clinical impact, and cost-effective therapies are already widely used. Clinical trials should only proceed when a sound argument around its ultimate competitive advantage in a given medical/surgical condition is clearly articulated.
Subject Selection
Recommendation 3.4.2.4: Individuals who participate in clinical stem cell research should be recruited from populations that are in a position to benefit from the results of this research. Groups or individuals must not be excluded from the opportunity to participate in clinical stem cell research without rational scientific justification. Unless scientifically inappropriate, trials should strive to proportionally include women, as well as men, and members of all ethnic groups.
Well-designed clinical trials and effective stem cell-based therapies should be accessible to patients without regard to their financial status, insurance coverage, or ability to pay. In stem cell-based clinical trials, the sponsor and principal investigator should make reasonable efforts to secure sufficient funding so that no person who meets eligibility criteria is prevented from enrollment because of their inability to cover the costs of participation.
Assuming that a particular condition is not thought to adversely affect decision-making capacity, clinical research should generally seek to enroll those who have a capacity to provide consent rather than those who are unable. In some cases, first-in-human trials might be started in children because they are the only disease-affected individuals who might benefit from the intervention. When conducting late-phase or post-approval trials, investigators should generally plan, design, analyze, and report trials to examine relationships between treatment response and age, sex/gender, or self-selected ethnic group.
Informed Consent
Recommendation 3.4.2.5: Informed consent must be obtained from potential human subjects or their legally authorized representatives. Reconsent of subjects must be obtained if substantial changes in risks or benefits of a study intervention are identified or alternative treatments emerge during the research.
Culturally and linguistically appropriate counseling and voluntary informed consent are necessary components in the ethical conduct of clinical research and the protection of human subjects. Subjects should be made aware that their participation is voluntary. Patients who decide not to participate in clinical research should be reassured that they will continue receiving ongoing clinical care. In addition, consent discussions should emphasize that once the stem cell intervention is provided, it cannot be removed and that subjects must be free to withdraw consent for follow up without penalty at any point. Subjects should be informed that the investigational stem cell intervention may prevent them from receiving other therapies or participating in future clinical studies. Specific consent challenges in early phase trials are discussed below.
Recommendation 3.4.2.6: When human research participants lack the capacity to provide valid informed consent, when no other reasonably effective options exist, and the risks from study procedures should be limited to no greater than a minor increase over the minimal risk unless the risks associated with the intervention are exceeded by the prospect of therapeutic benefit. A legally authorized representative or substitute decision-maker should help make decisions that are in the patient’s interest.
Stem cell-based clinical trials may involve populations, such as children or persons with advanced neurological disorders, who may lack knowledge, comprehension and decision-making capacity required to provide informed consent. Because such individuals cannot make their own decisions and protect their own interests, they require extra protection from research risk. Most jurisdictions provide guidance concerning which legally authorized representatives or substitute decision-makers should be approached when prospective research participants lack decision-making capacity. This recommendation pertains to risks that lack a therapeutic justification, for example, tissue biopsies to test biodistribution, sham procedures, or withdrawal of standard treatments to monitor response during unmedicated periods. Such procedures should not exceed a minor increase over the minimal risk when trial populations lack capacity to provide valid informed consent. In addition, in this setting, the assent of the research subject should be obtained where possible even when informed consent cannot be obtained. Because definitions of minimal risk vary by jurisdiction, researchers should adhere to policies defined by local human subjects review committees.
The issue of obtaining informed consent and assent from children for research is not unique to stem cell research. Accordingly, research conducted with children, as with other individuals who lack the capacity to provide valid consent, should adhere to recognized ethical and legal standards for this research.
Assessment of Capacity to Consent
Recommendation 3.4.2.7: Prior to obtaining consent from potential adult subjects who have diseases or conditions that are known to affect cognition, their capacity to consent should be assessed formally.
Subjects who lack decision-making capacity or have medical conditions that can adversely affect decision-making capacity should not be excluded from potential biomedical advances involving stem cells. At the same time, patients who lack capacity should be recognized as especially vulnerable. Conclusions that individuals lack decision-making capacity should only be reached after formally assessing their capacity to provide consent. When individuals are deemed to lack decision-making capacity, as permissible by law and following established ethical guidelines, steps should be taken to involve legally authorized representatives who are qualified and informed to make surrogate research judgments. See also Recommendation 3.4.2.6.
Privacy
Recommendation 3.4.2.8: Research teams must protect the privacy of human subjects.
Privacy is an important value in clinical settings. Moreover, there are longstanding professional obligations and legal duties to maintain confidentiality in medical care and research. Given the high profile of many stem cell-based intervention trials, it is particularly important for research teams to take steps to protect the privacy of research subjects. For instance, research data should be maintained in a secure manner with access restricted to study staff, oversight bodies, and agencies who have a legitimate right and have undergone training in management of private data to review these data as would be the case in any clinical trial.
Patient Sponsored and Pay-to-Participate Trials
Recommendation 3.4.2.9: Patient-sponsored and pay-to-participate trials pose challenges for ensuring scientific merit, integrity, and priority as well as fair selection of study participants. Accordingly, charging individuals to participate in clinical trials should only be permitted when such studies are compliant with applicable national regulations and are approved and supervised by a rigorous independent review body, such as an institutional review board.
As a general rule, study participants should not be charged to access investigational products or to participate in clinical studies. Exceptions to this rule should be subjected to close scrutiny by responsible parties such as institutional review boards and national regulators. The review process for pay-to-participate trials should ensure compliance with the principles outlined in these guidelines regarding the integrity of the research enterprise, transparency, and patient welfare. The process should consider all fees study participants are expected to pay and determine whether there is any credible basis for charging fees to individuals enrolled in the clinical trials. With studies that require authorization or clearance by national regulators, such regulators should be informed that study participants will be charged. They must then determine whether any and all fees charged to study participants comply with ethical, legal, and scientific standards. The potential liabilities of patient-sponsored and pay-to-participate research should be managed by requiring that protocols considering the use of such arrangements undergo independent expert review for scientific rationale, priority, and design. While input from patient communities can greatly enhance the research process, independent oversight is essential to ensure the responsible conduct of research and its reporting. Oversight bodies such as institutional review boards and research ethics committees must examine ethical, scientific, and legal features of pay-to-participate studies, ensuring they comply with applicable regulations and contemporary standards for research ethics.
Whereas patient advocacy and disease groups interested in funding clinical studies may have a strong research orientation and have the capacity required to carefully assess ethical, legal, and scientific issues related to designing and conducting clinical trials, individual patients seeking trial access may not have the resources or background needed to evaluate the ethical and scientific implications of charging research participants for access to investigational products administered in clinical studies. Consequently, patient payers, however well-intentioned, may press for studies that are poorly justified, are not well designed, or blur the lines between treatment and research and promote therapeutic misconception or other misunderstandings that undermine meaningful informed consent. Pay-to-participate research also raises questions of selection bias given that only those with access to resources may be able to enroll in trials and bias for participation in the treatment vs. the placebo group.
Patient-sponsored trials present opportunities for individuals and groups of patients to directly engage in the research process and fund work that public and industry sponsors are unwilling to undertake. Nevertheless, they present serious ethical and policy challenges that need to be addressed. Patient sponsors may press for study designs that eliminate elements such as randomization to a comparator arm and eligibility criteria that are critical for promoting scientific validity and patient welfare. Patient sponsors may also lack the expertise to distinguish meritorious protocols from those that are scientifically dubious. Further, there may be confusion over the intellectual property rights associated with successful interventions. Finally, they can also have the effect of diverting prospective study participants from studies that are well-designed and have the potential to generate meaningful safety and efficacy data to trials with serious methodological shortcomings.
Pay-to-participate studies also raise ethical concerns that are not confined to the study subjects who wish to enroll in such trials. By potentially coopting research teams from pursuing research endeavors that have received support through more traditional peer-reviewed mechanisms, pay-to-participate studies can result in outcomes that may unfairly disadvantage patients who lack the financial resources to set research agendas. In addition, patient-sponsored trials may divert resources such as study personnel from research activities that advance more promising research avenues.
Finally, because patients transact directly with those offering trial participation, direct payment for participation supports a business model whereby patients might be charged for receiving unproven and ineffective stem cell-based interventions and feel under pressure to accept such interventions from those “selling” it.
-
Registration
Recommendation 3.4.3.1: All trials should be prospectively registered in public databases.
Registration offers transparency regarding promising stem cell-based interventions so that patients, regulators, and the scientific community can monitor these efforts and incorporate them into future efforts, thereby minimizing risk and maximizing benefits of clinical trials. Registration also promotes integrity in scientific research, such as ensuring that scientists do not change primary endpoints after studies commence or otherwise take steps that might compromise the quality of research data. In addition, registration promotes access to clinical trials for patients who might not otherwise have a means of knowing about them. However, having a trial listed on public databases does not necessarily mean that the trial has been vetted by regulators or is in compliance with these guidelines. Prospective patients or their representatives should always confirm that trials are authentic before enrolling.
Adverse Event Reporting
Recommendation 3.4.3.2: Investigators should report adverse events, including their severity and potential causal relationship with the experimental intervention.
Knowing the safety profile of stem cell-based interventions is critical for effective translation. Timely analysis of safety information is also crucial for reducing the uncertainties surrounding stem cell-based interventions. Unfortunately, many studies report deficiencies in adverse event reporting for novel therapeutics (Saini et al., 2014). Researchers should report adverse events associated with cells, procedures, and all other aspects of the intervention. Most if not all national regulatory agencies mandate the reporting of such events, as they would for any clinical trial, and thus trials of stem cells should do the same at all trial phases.
Publication
Recommendation 3.4.3.3: Researchers should promptly publish results regardless of whether they are positive, negative, or inconclusive. Studies should be published in full and according to international reporting guidelines, including registration in the public databases.
Publication of all results and analyses, regardless of whether an agent is advanced to further translation or abandoned, is strongly encouraged to promote transparency in the clinical translation of stem cell-based therapies, to ensure the development of clinically effective and competitive stem cell-based therapies, to prevent individuals in future clinical trials from being subjected to unnecessary risk, and to respect research subjects’ contribution (Fung et. al 2017). As such, reporting must be timely and accurate with the ambition to report long term follow up as well for those therapies where the agent is predicted to survive long term. Publication of data without paywalls is encouraged. Researchers should also consider ways to share individual research subject data, provided that adequate privacy protections for research subjects can be assured. A U.S. Institute of Medicine Report offers principles on sharing clinical trial data (Institute of Medicine, 2015). Researchers, sponsors, and others should adhere to these principles. Additional information is also available from the AllTrials initiative (https://www.alltrials.net), which the ISSCR supports.
If a particular project can be described according to internationally recognized reporting guidelines, this format should be used. For example, researchers should report all randomized trials according to the CONSORT statement recommendations (Consolidated Standards of Reporting Trials; http://www.consort-statement.org/). Journal editors should accommodate publication of inconclusive and disconfirmatory findings. See also Section 4, Communications. Publications should also be included in the clinical trial registries to allow easy access to the outcome of the trial.
-
Early phase trials provide the first opportunity to evaluate the methods and effects of promising stem cell-based interventions in humans. They also represent the first occasion where humans are exposed to an unproven intervention. All preclinical study results, including negative and neutral studies, should be considered before first in human trials are started. Because early phase studies of stem cell-based interventions involve high levels of uncertainty, investigators, sponsors, and reviewers may have very different views about the adequacy of preclinical support for trial initiation.
Consent in Early Phase Trials
Recommendation 3.4.4.1: Consent procedures in any prelicensure phase, but especially early phase trials of stem cell-based interventions, should work to dispel potential research subjects’ overestimation of benefit and therapeutic misconception.
Early phase trials involving stem cell-based interventions may enroll research participants who have exhausted standard treatment options. In some cases, trials enroll individuals who have just experienced a life-altering medical event, such as spinal cord injury. Such individuals may be prone to overestimating the likelihood or degree of benefit of the experimental intervention (“therapeutic misestimation”). Individuals may further perceive research procedures as having a therapeutic benefit (“therapeutic misconception”). Both therapeutic misestimation and misconception may lead individuals to inadequately weigh risks of study participation, including health, social, logistic, and economic risks. Both may derive from overly optimistic reporting on stem cell research in traditional and new/social media. Accordingly, investigators should adopt a position of therapeutic equipoise, be aware of media/public representations of their field and make particular efforts to ensure that informed consent is valid in this setting (Benjaminy et.al 2015). Approaches that might be considered include:
Conducting informed consent discussions that include someone who is independent of the research team.
Explaining that major therapeutic benefits in early phase studies are exceedingly rare and that there may be unknown side effects given that the intervention has not been tried in people before.
Addressing misconceptions and misestimations that derive from public representations of the field.
Testing the comprehension of prospective participants about risks and benefits before accepting their consent to allow for the relevant data to be read and understood with the opportunity to ask questions about it.
Requiring a period between consent discussions and acceptance of consent.
Avoiding language that has therapeutic connotations, for example, using words like agent or cells or intervention rather than “stem cell therapy” or “treatment.”
Supplementing consent forms with additional educational materials.
Resources for drafting consent forms in early phase trials can be found at the National Institutes of Health Office of Biotechnology Activities (National Institutes of Health, 2014).
Sequence of Testing
Recommendation 3.4.4.2: In general, initial tests of a novel strategy should be tested under lower-risk conditions before escalating to higher risk study conditions even if they are more likely to confer therapeutic benefit.
The approach of risk escalation enables researchers to refine and test techniques before advancing towards more aggressive strategies. It also helps to minimize the prospect of catastrophic events that might undermine confidence in development of stem cell-based interventions. Investigators should generally begin at lower doses, use less risky delivery procedures, use less aggressive co-interventions, and stagger treatment but also not use doses that are unlikely to have any therapeutic effect for the patient. Staggered treatment provides the opportunity to carefully review experiences and results prior to posing risk to additional subjects. A clear plan on how this will be done is needed in terms of the process by which a decision on dose changes will be undertaken. Researchers should, in general, validate safety and techniques in research subjects with advanced disease before testing their products in research subjects with more recent disease onset. There may nevertheless be situations where, because of delivery or disease target, a cell product is not suitable for initial evaluation in individuals with advanced disease.
Maximizing Value
Recommendation 3.4.4.3: Researchers should take measures to maximize the scientific value of early phase trials.
Many interventions tested in early phase trials do not eventually show efficacy. However, even unsuccessful translation efforts return a wealth of information for developing stem cell-based interventions. Researchers should take several steps to maximize what is learned in early phase trials. First, where possible they should design studies that identify dose effects and mechanisms of action. These help researchers to determine whether cells have worked in the way anticipated. Second, they should seek to use standardized assays, endpoints, and methods. This enables researchers to synthesize results from individual, statistically underpowered trials (see Recommendation 5.1). Third, researchers should publish trials, methods, and sub-analyses in full. Studies show that many aspects of early phase studies are incompletely reported (Camacho et al., 2005; Freeman and Kimmelman, 2012). Last, where resources permit, and with appropriate consent, researchers should bank tissues and approach research subjects or families for permission to perform an autopsy in the event of death (see also Recommendation 3.4.6.3).
-
Late phase trials are aimed at providing decisive evidence of clinical utility. They do this by using clinical measures of benefit, typically in larger numbers of participants, and by monitoring response over a longer, more clinically relevant period. Late phase trials generally use randomization and comparator arms to protect the ability to draw valid conclusions about clinical benefit. The choice of comparator presents some distinctive ethical challenges in the context of stem cell-based interventions. When designing late phase clinical trials, researchers should select objective and measurable primary endpoints (clinical and validated surrogate endpoints).
Choice of Comparators
Recommendation 3.4.5.1: Clinical research should compare new stem cell-based interventions against the best therapeutic approaches that are currently or could be made reasonably available to the local population.
Stem cell research is an international endeavor where local standards of care differ dramatically. Due consideration should be given to achieve the best care in a given country, taking into consideration legitimate factors that affect the quality of care available locally. Trials should not be conducted in a foreign country solely to benefit patients in the home country of the sponsoring agency. Similarly, trials should not be conducted in a foreign country solely due to less stringent regulation. The test intervention, if approved, should realistically be expected to become available to the population participating in the clinical trial through existing health systems or those developed on a permanent basis in connection with the trial. In addition, research should be responsive to the health needs of the country in which it is conducted. For example, clinical trials with comparator arms should compare new stem cell-based interventions against the best therapeutic approaches that are currently available to the local population.
Placebo and Sham Comparators
Recommendation 3.4.5.2: Where there are no proven effective treatments for a medical condition and stem cell-based interventions involve invasive delivery, it may be appropriate to test them against historical controls, placebo, or sham comparators, assuming early experience has demonstrated the feasibility and safety of the particular intervention.
Once early phase trials appear to demonstrate feasibility and tolerability/safety, the next phase 2/3 trials should be designed to show safety and efficacy, as well as superior treatment to standard of care for the respective disease or at least equivalence with a safety and a cost-effective advantage. In order for this to be done, stem cell-based interventions should be tested as for any other therapeutic agent and include control subjects. In some cases, historical data from the subject or the patient population may be suitable. If historical data does not provide a suitable control, including a placebo or sham arm or in exceptional circumstances, a therapeutic comparator may be justified. In all such cases, the choice of a control arm should be explicitly justified. For the cellular products that require surgery to be administered, it is important to investigate the feasibility of blinding carefully, taking into account invasiveness and ethics of sham surgery. If blinding of sham surgery is unfeasible, considering other strategies to enhance blinding, such as blinding the evaluators, is essential.
Obviously, some sham procedures are not without risks, e.g. surgery. However, the use of sham comparators might be needed to assess the therapeutic potential of the intervention, but this can only be realistically done when issues around dosing and delivery have been resolved and felt to be optimized. In addition, researchers should ensure that the validity and advantages of sham procedures are not undone by factors that could unblind research subjects or investigators. Maintaining blinding can be particularly challenging in an era when research participants are able to use social media platforms to locate one another and communicate about their experiences as study subjects.
Researchers should also take particular care explaining the use of placebos or sham procedures during the informed consent process and ensure patients understand and agree that they may receive a treatment with no anticipated clinical benefit and that this may tie them into a trial for years.
-
Data Monitoring
Recommendation 3.4.6.1: A data-monitoring plan is required for clinical studies. When deemed appropriate, aggregate updates should be provided at predetermined times or on-demand. Such updates should include adverse event reporting and ongoing statistical analyses if appropriate. Data monitoring personnel and committees should be independent from the research team.
The risk/benefit balance can change over the course of clinical research, as safety and response are observed, recruitment wanes, or as new treatments become available. This is especially true for stem cell-based intervention trials, which are characterized by high uncertainty and rapidly evolving science. The welfare of subjects must be carefully monitored throughout the duration of stem cell-based clinical trials, the study interrupted if the risk/benefit balance becomes favorable or unfavorable, and subjects informed of new information about themselves, the trial, or the intervention that might materially affect their continued participation in a study.
Long-term Follow-up
Recommendation 3.4.6.2: Given the potential for transplanted cellular products to persist indefinitely and depending on the nature of the experimental stem cell-based intervention, subjects should be advised to undergo long-term health monitoring. Long-term follow-up is mandated in some countries, often for the use of gene therapies or xenotransplants. Additional safeguards for ongoing research subject privacy should be provided. Subject withdrawal from the research should be made in an orderly fashion to promote physical and psychological welfare.
Long-term follow-up provides an opportunity to monitor the emergence of late adverse events and the durability of benefit. Given the practical realities, conducting long-term follow-up may be challenging. Investigators should develop and adopt measures to maintain contact with research subjects. In addition, funding organizations should be encouraged to develop mechanisms for supporting long-term follow-up. Since the length of appropriate follow-up is impossible to specify in the abstract, the decisions about this should be clearly articulated by investigators and reviewed by independent peer-reviewers and oversight bodies. If subjects withdraw from a study after the product has been delivered, investigators should continue long-term follow-up to monitor the emergence of adverse events if subjects concur.
Autopsy
Recommendation 3.4.6.3: To maximize the opportunities for scientific advance, research subjects or surviving next of kin in stem cell-based intervention studies should be asked for consent to a partial or complete autopsy in the event of death to obtain information about cellular implantation and functional consequences at some point in the trial. Requests for an autopsy must consider cultural and familial sensitivities and be conducted in a respectful and compassionate manner. Researchers should strive to incorporate a budget for autopsies in their trials and develop a mechanism to ensure that these funds remain available over long time horizons.
Though a delicate issue, access to post-mortem material substantially augments the information coming out of trials and enables future product or delivery refinements in the treated condition. Since consent for autopsy is typically obtained from the family members of someone who has died, investigators should facilitate discussion of this issue among subjects and appropriate family members well ahead of any anticipated terminal event.
-
Recommendation 3.4.7.1: The clinical use of genetically altered (including genome-edited) somatic stem cells should be reserved for the treatment or correction of severe disease and disability. Due to the inherent risks, these products should comply with established policies and regulations for genome editing and cell-based products.
Potential clinical applications of genetically altered somatic stem cells should be evaluated for potential risks and benefits of serious medical diseases and conditions. Uses of genetic alteration for non-serious conditions or for enhancement of body performance or features, such as to give an advantage in sports, should be discouraged: the potential benefits are marginal and cannot offset the risks at this time; they are unlikely to have public support; and they could bring the field into disrepute. The current risks associated with the methods also make it inadvisable to use them in attempts to confer disease resistance.
The genetic alteration of cells provides a potential long-term/lifetime treatment for certain diseases. However, there are risks associated with this approach that include:
Off-target effects from the insertion of exogenous DNA in gene replacement applications.
Incorrect on-target and off-target genetic events in genome editing applications.
Large-scale chromosomal rearrangements/inversions due to multiple DNA cleavage events when using targeted nucleases in genome editing.
Unwanted immune response to the virus or nucleic acids from the viral vector carrying the DNA or exogenous DNA.
A detailed discussion of these and other issues around clinical trials with genome-edited stem cells can be found in Appendix 5.
-
Mitochondrial Replacement Techniques
Recommendation 3.4.8.1: Mitochondrial Replacement Techniques (MRT) should be offered only in the context of clinical investigation that is subject to strict regulatory oversight, limited to patients at high risk of transmitting serious mitochondrial DNA-based diseases to their offspring, when no other treatments are acceptable, and where long-term follow-up is feasible. International data sharing arising from initial uses is essential to help inform the field and ensure its appropriate use.
Initial applications of MRT should continue to be restricted to cases in which the probability of transmission of pathogenic mitochondrial DNA is very high, where preimplantation genetic testing is unlikely to identify embryos suitable for transfer, and where procedures are conducted in the context of clinical investigation that can contribute to generalizable knowledge about this currently unproven and experimental technique. Preclinical research in human embryo-derived stem cells following MRT indicated the possibility of levels of maternal mitochondrial DNA increasing with extended passaging, but the clinical relevance of these data is unclear. Concerns have also been expressed about the possibility of mito-nuclear interactions being disrupted by MRT, though these remain theoretical. Research on embryonic stem cells derived from embryos after MRT or on the embryos themselves maintained in vitro would help explore these issues. These experiments could only proceed in jurisdictions where the creation of embryos for research is allowed, and only where they have been permitted following review by a specialized oversight process (see Section 2.1).
Recommendation 3.4.8.2: There are inadequate clinical and preclinical data to justify the use of MRT to treat unexplained infertility associated with poor oocyte/embryo quality in women; therefore, it is recommended that this not be an intervention at this time.
MRT has been used in the clinic as a speculative treatment for infertility (Zhang et al 2016). Given the risks entailed and the absence of clear mechanisms and compelling rationale for the use of MRT for unexplained infertility, additional preclinical and clinical experience are required to establish safety and efficacy. In one reported pilot trial of MRT, embryonic development and pregnancy rates in patients of advanced maternal age did not increase (n= 30), and it was advised that such patients should not undergo MRT (Mazur, 2019). Data from another small (non-randomized) trial of MRT (n= 25), in women under 40 with previous multiple failures of IVF (Costa-Borges et al, 2020), suggest that further controlled trials and follow-up are required. Research conducted in vitro to understand the mechanism by which application of the techniques may help unexplained infertility (which might not involve mitochondria) should be conducted, notably because this may lead to alternatives that both circumvent the use of technically challenging methods and avoid any risks associated with heteroplasmy or disrupted mito-nuclear interactions.
Heritable Genome Editing
Recommendation 3.4.8.3.1: Substantial preclinical research is needed to minimize the potential harm associated with clinical applications involving heritable genome editing; therefore, any attempt to modify the nuclear genome of human embryos for the purpose of reproduction is premature and should not be permitted at this time (see Section 2.2.3A, Category 3A, a).
Any decision to proceed with heritable genome editing, where modified human embryos are transferred into a uterus or otherwise allowed to develop in utero, must be preceded with adequate preclinical research to minimize the potential harms from intended and unintended edits (see Recommendation 2.1.4). The first-in-human clinical uses should only be considered for the most favorable balance of potential harms and benefits and this will be most clearly defined for diseases and patients for which there are no viable alternatives. This may include prospective parents for whom there are no or very limited available alternatives for preventing transmission of diseases and conditions for which mortality is high and morbidity is severe. Other options for having a healthy child, including adoption, gamete or embryo donation, and preimplantation genetic testing, should be considered with appropriate counselling prior to any decision to proceed.
Recommendation 3.4.8.3.2: If the technical and safety challenges associated with human heritable genome editing are resolved (see Recommendations 2.1.4 and 3.4.8.3.1), any applications for the initial clinical use of human heritable genome editing should be evaluated on a case-by-case basis. This evaluation needs to consider not just the scientific methods, but also the societal and ethical issues associated with the proposed use.
The decision to proceed with first-in-human clinical uses needs to be taken openly with robust consideration of informed public opinion generated through meaningful public engagement. In addition, and critically, any experimental use of heritable human genome editing should only proceed in jurisdictions with appropriate and robust regulations and oversight.
A key consideration of potential uses of heritable genome editing is whether the prospective parents have feasible options for conceiving a genetically related child who does not inherit a serious genetic disease, such as preimplantation genetic testing and selection of embryos. The initial uses should be confined to prospective parents who lack reasonable alternatives.
It is important that the biological consequences of the intended genome edit are well understood, both for the immediate offspring and for future generations who might inherit it, in order to minimize the potential for an intended edit to have unintended deleterious consequences (on its own, via genetic interactions with other loci, or via environmental interactions). At present, the best way to achieve this goal is to use editing to change a known pathogenic genetic variant to one that is present in unaffected family members, common in the relevant population, or known not to be disease-causing.
Recommendation 3.4.8.3.3: A comprehensive regulatory and ethical framework for overseeing heritable genome editing must be established before any first-in-human clinical applications are considered. This framework should build on the existing regulatory frameworks for new biotechnologies, the practice of medicine, and the principles outlined in these guidelines (see Section 3.3 and 3.4).
The regulatory framework for heritable genome editing must ensure that there is robust multi-generational follow-up to identify adverse reactions that may occur due to inherited genome alterations. However, this needs to be done in such a way as to protect the confidentiality of the prospective parents and any children born. The framework must ensure that there is a robust informed consent process that builds on the informed consent process discussed in these guidelines (see recommendations 3.4.2.5 and 3.4.4.1) and includes a discussion of potential alternative treatments (if any) and the multigenerational risks and benefits of pregnancies involving the implantation of germline genome edited embryos, including those derived from genetically modified gametes.
Recommendation 3.4.8.3.4: Regulators, research funders, and academic and medical societies should seek to prevent the premature or unethical clinical uses of heritable genome editing unless and until the safety, ethical, and societal issues associated with the clinical use of heritable genome editing are resolved.
It is incumbent upon the entire biomedical research community to monitor for potential unethical and premature clinical uses of human heritable genome editing technologies. Researchers are strongly encouraged to report potential unethical uses to regulators, funders, licensing bodies, and academic societies to evaluate potential unethical uses of this technology.
-
In utero administration of a stem cell-based or gene-based intervention (whether based on gene replacement or genome editing) may offer several advantages, including 1) early intervention before tissue damage is established and when the tissue/cells have the highest growth and regeneration potential; 2) more effective bio-distribution of the intervention within the intended tissue while interstitial diffusion is facilitated, tissue barriers are still immature, and more comprehensive modification of the target cell population is possible because of its smaller size; and 3) low risk of eliciting immune response to the stem cell-based or gene product, because of the incomplete development of the adaptive immune system.
Considerations for in utero Genome Editing Interventions
While there may be therapeutic advantages, in utero genome editing interventions may also exacerbate some safety concerns, particularly those associated with genetic interventions. Early and more comprehensive exposure to gene transfer/editing techniques may increase the risk of genotoxicity, because of the high rate of cell proliferation and tissue growth and increased proportion of self-renewing progenitors. The broad biodistribution of the therapeutic product may also reach unintended tissues or cell populations that are otherwise shielded at older ages, such as germline cells. Finally, any acute or delayed toxicity triggered by the administered cell/gene product at the target and off-target tissues may have far more damaging consequences than observed when genome editing is performed at later stages of life, including teratogenicity. Ad hoc comprehensive studies should thus be designed in surrogate small and large animal models to assess these risks and investigate any long-term consequences of the intervention.
Recommendation 3.4.9.1 Clinical research involving in utero stem cell-based interventions or genome editing involves risks to both the pregnant woman and the future child, and should be undertaken only when it offers the prospect of a benefit greater than that of post-natal interventions, does not pose excessive risk to the pregnant woman, and where there is institutional capacity for autopsy (in the case of miscarriage or stillbirth) or follow-up (in the case of live birth).
Clinical research involving in utero genome editing or stem cell-based interventions should only be performed in centers with personnel trained for in utero surgery and with existing guidelines or practices regarding the treatment of extreme preterm births or births of children with devastating/life-threatening conditions. Research protocols for experimental in utero interventions must be reviewed and approved by a research oversight committee prior to recruiting patients. The interventions should be conducted as early in pregnancy as medically appropriate in case termination is unexpectedly needed due to risks to maternal health or the prospect of miscarriage, stillbirth or neonatal condition inconsistent with survival. While there is a risk of pregnancy complications after fetal intervention, the anticipated prospect of benefit from the intervention should be greater than the risk of complications in experienced hands.
Furthermore, the pregnant woman should be competent and able to voluntarily choose or refuse the intervention. The consent process should include a full discussion of alternative post-natal therapeutic interventions, as well as the possibility that even if this prenatal intervention is successful, there might nonetheless be a miscarriage, a stillbirth, or a child born with serious health problems. If permitted by the pregnant woman or required by law, the intended rearing partner should be consulted.
-
The ISSCR condemns the administration of unproven stem cell- and other cell- and tissue-based interventions outside of the context of clinical research or medical innovation that is compliant with the guidelines in this document (see recommendation 3.5.2), particularly when it is performed as a business activity. Scientists and clinicians should not administer unproven interventions outside of clinical research or medical intervention as a matter of professional ethics. For the vast majority of medical conditions for which putative “stem cell therapies” or “regenerative therapies” are currently being marketed, there is insufficient evidence of safety and efficacy to justify routine or commercial use. Serious adverse events subsequent to such interventions have been reported and the long-term safety of most stem cell-, cord blood-, bone marrow-, and other cell-based interventions (i.e., mesenchymal stromal cells) remains undetermined. The premature commercialization of unproven stem cell interventions, and other cell-based interventions inaccurately marketed as “containing,” “acting on,” “derived from,” or “like” stem cells, not only puts patients at risk but also represents a serious threat to legitimate stem cell research. Widespread marketing and clinical use of unproven cell or tissue-based interventions reduces the number of individuals able to participate in credible clinical studies, risks jeopardizing the reputation of the field and causes widespread confusion about the actual state of scientific and clinical development.
Recommendation 3.5.1: The clinical use of unproven stem cell-based interventions should be limited to well-regulated clinical trials and medical innovations compliant with these guidelines (Recommendation 3.5.2) and local laws, policies, and regulations. Government authorities and professional organizations should establish and strictly enforce policies and regulations governing the commercial use of stem cell based medical interventions.
Historically, many medical innovations have been introduced into clinical practice without a formal clinical trials process. Some innovations have resulted in significant and long-lasting improvements in clinical care, while others have subsequently been demonstrated to be ineffective or harmful. Stem cell-based interventions typically entail complex manufacturing protocols that should rarely, if ever, be developed outside a formal clinical trials process. Nonetheless, in some very limited cases, clinicians may be justified in attempting medically innovative stem cell-based interventions in a small number of seriously ill patients. Although attempting medically innovative care is not research per se, it should not be embarked upon unilaterally. It is incumbent upon the clinician to obtain scrutiny by external experts through peer review, institutional oversight, and presentation of observations and data in peer-reviewed medical publication so that the knowledge can benefit all. Such limited attempts at medical innovation contrast with the advertisement, sale, and administration of unproven stem cell interventions.
Hospital Exemption
Regulators in some countries provide a “hospital exemption” to enable individualized care for patients. This exemption from requirements for regulatory evaluation of safety and effectiveness is only appropriate when the risks of the intervention are low and consistent with the risks typically associated with conventional surgical or medical procedures. Furthermore, the existence of such narrow exemptions should not be used as a vehicle for providing unapproved stem cell-based interventions or avoiding regulatory oversight, as occurs when cell-based interventions requiring pre-marketing authorization are inaccurately promoted as being exempt from regulatory scrutiny and approval requirements. Given the potentially serious risks associated with substantially manipulated tissues and cells and non-homologous uses, and the need to study their effectiveness, it is important that such interventions and uses be ineligible for these regulatory exemptions. In jurisdictions that provide hospital exemptions and have not established well-defined criteria that limit the scope of the exemption, regulators are urged to narrowly define them as including only low risk, minimally manipulated cells and tissues for homologous use.
Surgical Procedure Exemption
Regulators also often provide a narrow “same surgical procedure exemption,” excluding tissue- and cell-based interventions from certain regulatory requirements when cells or tissue are collected from, and delivered to, the same patient during the same procedure. These exemptions should be narrowly crafted to allow common surgical procedures like skin grafts, while excluding tissue and cell preparations that have been substantially manipulated or are being provided for a non-homologous use. This pathway should not be used to provide experimental and unapproved stem cell-based interventions.
Stem Cell Based Medical Innovation
Recommendation 3.5.2: Given the many uncertainties surrounding medical innovations involving stem cells and their direct derivatives, this pathway is rarely ethically and scientifically justifiable and should be limited to a very small number of patients and restricted to a) the off-label use of authorized therapies (see Recommendation 3.5.3), b) unproven interventions provided through expanded access pathways (see Recommendation 3.5.4), or c) minimally manipulated stem cell based interventions for homologous uses. Such interventions should only be provided to patients according to the highly restrictive provisions outlined in this section and the other referenced recommendations.
The written plan for the procedure must include:
Scientific rationale and justification explaining why the procedure has a reasonable chance of success, including any preclinical evidence of proof-of-principle for efficacy and safety. Explanation of why the proposed stem cell-based intervention should be attempted rather than existing treatments. Description of how the cells will be administered, including adjuvant drugs, agents, and surgical procedures.
Plan for clinical long-term follow-up and data collection to assess the effectiveness and adverse effects of the cell-based interventions.
The written plan is approved through a peer review process by appropriate experts who have no vested interest in the proposed procedure.
The written plan is approved by an independent oversight body after evaluating the risks and benefits for patients. In the academic context this would be routinely done through an institutional review process for human subjects research.
The patient is not eligible for an existing stem cell-based trial for this indication.
The clinical and administrative leadership of the healthcare institution supports the decision to attempt the medical innovation and the institution is held accountable for the innovative procedure.
All involved personnel have appropriate qualifications and training, and the institution where the procedure will be carried out has appropriate facilities and processes of peer review and clinical quality control monitoring.
Voluntary informed consent is provided by patients according to the ISSCR Informed Consent Standard (see Appendix 6).
There is an action plan for addressing adverse events that includes timely and adequate medical care and if necessary psychological support services.
Insurance coverage or other appropriate financial or medical resources are provided to patients to cover any adverse events arising from the intervention.
There is a commitment by clinician-scientists to use their experience with individual patients to contribute to generalizable knowledge. This includes:
Ascertaining outcomes in a systematic and objective manner
A plan for communicating outcomes, including negative outcomes and adverse events, to the scientific community to enable critical review (for example, as abstracts to professional meetings or publications in peer-reviewed journals).
Initiating a formal clinical trial for the intervention in a timely manner after experience a very small number of patients.
Off-label Use
Recommendation 3.5.3 Off-label uses of stem cell-based interventions should be employed with particular care, given uncertainties often associated with off-label uses generally and associated with stem cell-based interventions specifically.
Physicians generally may use approved drugs and biologics for indications or patient populations other than those for which they have been shown to be safe and effective. This practice is commonly known as providing products on an “off-label” basis. Such off-label applications, distinct from administering products for the purposes for which they have been studied and approved, as specified on their prescribing information and package labels, constitute a common aspect of medical practice. Nevertheless, they present distinct challenges for stem cell, tissue or cell-based interventions.
First, depending on the jurisdiction, some stem cell-based interventions are not authorized for a specific use due to exemption from premarketing approval requirements. This can limit physicians’ access to reliable information on validated uses. Second, the complex biological properties of living cells and the limited clinical experience with cell-based therapies present uncertainties about long-term safety and effectiveness. Physicians should therefore exercise particular care when administering stem cell-based interventions on an off-label basis. As a rule, off-label use should only be offered when supported by high quality evidence or in situations consistent with current scientific knowledge, applicable regulations and institutional policies, and the standards of the international medical community. Patients must be informed in advance if a proposed off-label use has not been evaluated for safety and efficacy with respect to their specific medical condition. Off-label use of stem cell products is likely to increase as more stem cell therapies obtain pre-marketing authorization for particular indications. Providing such interventions on an off-label basis will need to be done with great caution, attentiveness to the available evidence base, and with the informed consent of potential recipients.
As a general principle, physicians should conduct controlled, supervised studies to establish safety and efficacy for new applications of products or interventions that have been approved in a distinct clinical setting. As evidence of safety and efficacy accumulates, regulatory bodies are provided with the data they require to consider expanding the indications that fall within the scope of product labeling.
Pre-approval Non-trial Access to Experimental Stem Cell-based Interventions
Recommendation 3.5.4 Pre-approval access to experimental stem cell-based interventions should be limited to well-regulated programs that require prior authorization from national regulators.
Patients understandably sometimes seek experimental interventions when there are no established and approved treatments for serious or terminal diseases and conditions. Regulatory authorization for pre-approval non-trial access programs (often described as “expanded access”) provides important checks and balances to ensure patient safety, facilitates drug development, and preserves the integrity of clinical trials. In particular, national regulatory bodies sometimes have access to important information about risks associated with particular investigational interventions that is not always available to individual patients or institutional review boards.
-
Clinical translation continues after a product is taken into clinical practice. Realizing the full potential of a product requires gathering additional safety and efficacy evidence, controlling applications that lack complete evidentiary footing, and pricing products in a way that delivers value for patients and healthcare systems.
-
The regulatory review and approval process for stem cell-based interventions must rigorously evaluate each potential intervention to ensure the quality, safety, and efficacy of new treatments. Regulators should require substantial evidence from well-designed clinical trials to demonstrate that new products provide a clinically meaningful benefit for the target indication. The premature commercialization of stem cell-based interventions threatens the development of safe and efficacious evidence-based therapies and places unnecessary economic burdens on healthcare systems and the public.
Substantial evidence to establish effectiveness for market approval
Recommendation 3.6.1.1 The introduction of novel products into routine clinical use should be dependent on the demonstration of substantial evidence of effectiveness in appropriately powered, well-controlled clinical trials, with statistically significant findings.
Regulatory approval for commercialization represents a key pivot point in a product’s translation. National governments and regulatory authorities should maintain rigorous review pathways to ensure that stem cell-based products conform to the highest standards of evidence-based medicine. Early interactions and advice during the product development process may support the accelerated development of safe and effective new therapies.
Even after clinical studies of the highest standard have demonstrated safety and efficacy and regulatory approval pathways have been cleared, close attention must be paid to ensuring the safety and effectiveness of interventions that have entered routine or commercial clinical use. Further, the fairness of access should be consistent with local legal requirements and standards and the standards of ethical, evidence-based medicine. These standards include ongoing monitoring of safety and outcomes and ensuring accessibility by those who have the most pressing clinical need.
Accelerated Approval Pathways
Recommendation 3.6.1.2 When evaluating new interventions for rare diseases or life-threatening medical conditions, regulators should consider the acceptable balance of risk and clinical benefit appropriate to the medical condition and patient population for which new treatments are designed. All approval pathways should require substantial evidence of safety and effectiveness before products are marketed to patients.
Many countries already have well-defined accelerated approval pathways that can be adapted for stem cell-based products. These pathways may allow for the more regulatory interactions with product developers and the faster approval of products based on surrogate or intermediate endpoints that are reasonably likely to predict a meaningful clinical benefit.
Conditional Marketing Authorizations
Recommendation: 3.6.1.3 In jurisdictions with conditional approval mechanisms, regulators must ensure there is a robust post-market surveillance system whereby regulators have the capacity and power to remove products from the market as appropriate.
Regulators may need to make decisions about stem cell products based on limited safety and efficacy data (Bubela et al 2015). In terms of safety, the goal of many cell therapies is long-term engraftment, thus, side effects may only become apparent many years after the conclusion of clinical trials. For stem cell products targeting rare diseases, clinical trial size and duration may be inadequate to determine efficacy. Furthermore, randomized controlled trials for risky and invasive therapies may be prohibitively expensive and unethical from the perspective of participants enrolled in the control arm. Therefore, international regulators have made provisions for conditional marketing authorization and post approval studies to confirm safety and predicted efficacy. While post approval studies have the potential to provide additional data on safety and efficacy, product developers must continue to collect, analyze, and report safety and efficacy data to identify adverse events and confirm any therapeutic benefit of conditionally authorized products. When post approval studies are required, regulatory oversight bodies need to ensure they are conducted.
Considerations for Rare Diseases
Recommendation 3.6.1.4 In jurisdictions with existing approval pathways for orphan or rare diseases, those pathways should be used to facilitate the development of stem cell-based interventions.
Many jurisdictions have orphan disease designations for regulatory approval, because it is often difficult to ensure adequate statistical power in clinical trials for rare diseases. These pathways may accelerate access to approved stem cell therapies that have demonstrated safety and efficacy. In establishing orphan disease regulatory standards, jurisdictions should consider: the definition of orphan disease based on incidence (e.g., Japan – fewer than 50,000 patients in Japan; US – fewer than 200,000 in the US; Europe – prevalence is less than 5 in 10,000 Europeans). Jurisdictions generally limit the designation to serious, life-threatening, chronically debilitating disease, and unmet medical needs (no satisfactory authorized products). Further, in Europe and the US, it must be unlikely that the marketing of the product would generate sufficient returns to justify investment in its development. Jurisdictions provide a set of incentives that may include: tax credits for clinical testing, a voucher for accelerated approval for a different product, scientific advice and protocol assistance from regulators, a reduced fee for applications, priority review, potential coordination amongst regulators, internationally and extended periods of market exclusivity. Some jurisdictions may seek to recapture financial incentives if the therapy becomes highly profitable.
Bio- and Pharmacovigilance
Recommendation 3.6.1.5 Developers, manufacturers, providers, and regulators of stem cell-based interventions should continue to systematically collect and report data on safety, efficacy, and utility after they enter clinical use.
Stem cell-based interventions can remain biologically active for long periods and thus may present risks with long latencies. Additionally, stem cells and their derivatives can exhibit a range of dynamic biological activities and therefore be potentially difficult to predict and control. These may lead to pathologies including tumorigenesis, hyperplasia, and the secretion of bioactive factors that may exert secondary effects on physiological processes such as inflammation or immune response. Some types of stem cells are capable of migration after transplantation, meaning there is a risk of off-target effects and inappropriate integration. Further, tracking the locations of transplanted cells may be difficult using current technologies.
For these reasons, monitoring patients’ overall health status over the expected duration of the therapeutic benefit is critical and plans for the funding and conduct of long-term monitoring should be incorporated into study protocols early in the development of new interventions. These monitoring activities may include systematic post-market studies, event and outcome reporting by clinicians and patients, patient registries, and/or economic analyses of comparative effectiveness. The results of such monitoring activities should be promptly reported to regulatory authorities and the medical community.
Patient Registries
Recommendation 3.6.1.6 Registries of specific patient populations should be used to provide valuable data on the natural history and progression of diseases that can support the development of meaningful endpoints, biomarkers, and outcomes measures to facilitate the development of new products. Furthermore, patient registries are useful tools for monitoring adverse events after regulators have approved a product for routine clinical use. However, registries should not be substituted for well-regulated randomized controlled clinical trials designed to evaluate the safety and efficacy of complex products like stem cell and gene-based interventions.
Stakeholders in stem cell-based therapeutics, including researchers, physicians, regulatory bodies, industry, and patient and disease advocacy groups, should cooperate in developing disease history registries to facilitate the development of stem cell and gene-based products. Because these therapies are novel and may have some increased risk, continued surveillance of patient outcomes after the commercial launch of the cell or gene products is recommended. To this end, registries should be established to collect additional safety, efficacy, and durability data on stem cell and gene-based interventions after they have been approved for clinical use. As valuable as they are, such registries should complement randomized controlled trials and not be used as a substitute for them.
Biohacking
Recommendation 3.6.1.7 Provision and use of equipment and commercial kits for cell and gene-based interventions in humans should be limited to settings with an appropriate level of regulatory oversight to ensure their safe and responsible use.
Alongside the development of gene- and stem cell-based therapies has been an increased interest in self-administration and ‘do-it-yourself’ kits and equipment. Such “DIY” interventions are often promoted as a means of using “biohacking” to make improvements to personal health and well-being with little acknowledgment of risks posed by their use. Regulators and commercial providers should ensure that genetic alteration kits and equipment carry a warning that they are not approved for self-administration (e.g., SB-180, California State Legislature, 2019). Leaders in the emerging do-it-yourself biology movement are encouraged to continue to develop codes of practice based on these guidelines and other standards to inform best practice standards.
-
Support for stem cell research depends, in part, on its potential for advancing scientific knowledge, which may result in the development of clinical applications. As such, institutions, researchers, and providers in both the public and private sectors have a responsibility to promote public benefit, and specifically to ensure that research findings are accessible to the international scientific community and, importantly, equitable access to safe and effective therapies for those who need them. For these reasons, research, clinical, and commercial activities should seek to maximize affordability and accessibility.
Developer Consideration of Value
Recommendation 3.6.2.1: Stem cell-based interventions should be developed to deliver health and economic value to patients, payers, and healthcare systems.
Consideration of value and access should be built into research and development pipelines early to enhance the probability of market access in addition to regulatory market authorization. Following market authorization, product developers still need to seek positive coverage decisions from public and/or private payers. Many make coverage decisions based on health technology assessments (HTA). HTA is the process of considering synthesized evidence to arrive at a decision on whether a specific technology should be included in the portfolio of technologies provided by a specific health care system or covered by a specific health care payer. The recommendations are based on clinical and pharmacoeconomic evidence, cost-effectiveness or comparative-effectiveness data, patient perspectives, as well as ethical and implementation considerations. Most importantly, however, HTA recognizes opportunity costs within a payer’s health care budget. This means that money spent on one technology or service is not available to be spent on other technologies or services.
Many public health systems consider cost effectiveness, based on an incremental cost-effectiveness ratio (ICER). ICERs are comparative between existing and new therapies and are dependent on direct healthcare costs and changes in quality-adjusted life years (QALYs: life expectancy in years X quality of life). The level of the ICER threshold varies between countries and/or payers. Some payers have a differential ICER threshold for complex and specialist health care, which include Orphan Drugs.
Reimbursement and Payer Considerations
Recommendation 3.6.2.2: Payers, and healthcare systems should work with developers of stem cell interventions, patients, and regulators to establish processes to evaluate their health and economic value, including conditional pathways.
Recognizing the challenges in evidence generation faced by stem cell-based therapies, especially for rare diseases, payers in some jurisdictions are considering coordination of conditional reimbursement models with conditional regulatory approval models. These models rely on expanded powers of regulators for post-market oversight and infrastructure and systems for post-market surveillance, since evidence generation is shifted to varying extents to the post-market period. These approaches will rely heavily on the availability and quality of post-marketing data, and the associated analytic capacity. Further, alternative payment plans that amortize payments over time are under consideration, such as technology leasing arrangements or refunds, rebates or discounts if the technology does not provide the promised benefits, is effective for a shorter period-of-time than expected, or requires re-administration. Such complex funding arrangements are pre-determined; they are negotiated and enforced via managed access agreements.
The development and provision of clinical interventions are based on decisions made by patients, healthcare professionals, and payers. Key factors that influence such decisions include the known risks and benefits of available treatment options, individual preferences on the part of patients and treatment providers, and comparative availability and cost. Developers, manufacturers, and providers of stem cell-based interventions should recognize that, along with safety, efficacy, and accessibility, economic value is an important measure of the overall utility of any therapeutic. They should thus participate in studies intended to assess comparative effectiveness, particularly in countries in which such studies are legally mandated. Such studies involve the systematic comparison of currently available therapies for their full range of benefits and provide important information for medical decision-making.
Pricing
Recommendation 3.6.2.3: Developers, funders, providers, and payers should work to ensure that cost of treatment does not prevent patients from accessing stem cell-based interventions for life-threatening or seriously debilitating medical conditions.
Sponsors of research aimed at the development of stem cell-based interventions targeting seriously debilitating or life-threatening medical conditions should seek to support access to safe and efficacious therapeutics to any patient in need, irrespective of financial status. Post-trial access for individuals who participated in clinical research leading to the development of a licensed stem cell therapy is a particular priority.
Private firms seeking to develop and market stem cell-based interventions should work with public and philanthropic organizations to make safe and effective products available on an affordable basis to disadvantaged patient populations. Developers, manufacturers, and patient groups should engage with government regulators and health care funders to develop mechanisms for prompt and sustainable adoption of stem cell interventions for life-threatening or seriously debilitating medical conditions. Such mechanisms should balance the needs of those patients who will benefit with the responsibility of payers to the communities they serve and strengthen the evidence base for the safety, effectiveness, and long-term value of those therapies.
Return to TOC | 2021 Guidelines for Stem Cell Research and Clinical Translation
4. Communications
Stem cell research receives a great deal of attention from policymakers, the popular press, and popular culture, including social media. Given its scientific and clinical potential and the controversies that have surrounded the field, this high public profile is understandable. However, popular coverage and reporting in the medical literature are frequently far from ideal.
Return to TOC | 2021 Guidelines for Stem Cell Research and Clinical Translation
Stem cell research receives a great deal of attention from policymakers, the popular press, and popular culture, including social media. Given its scientific and clinical potential and the controversies that have surrounded the field, this high public profile is understandable. However, popular coverage and reporting in the medical literature are frequently far from ideal. The potential benefits of stem cell-based interventions are sometimes exaggerated and the challenges, including their clinical application and risks, are often understated. Inaccurate or incomplete representations can have tangible impacts on the expectations of the general public, patient communities, physicians, and on the setting of health and science policies. Companies and individuals marketing stem cells for unproven clinical uses exploit those inaccurate and incomplete representations.
Public Representation of Science
Recommendation 4.1: The stem cell research community should promote accurate, current, balanced, and responsive public representations of stem cell research.
The high level of public and media interest in the field provides stem cell scientists with ample opportunities to communicate their findings through a variety of popular and social media channels. The research community is encouraged to responsibly engage interactively with the public through outreach and communications and by providing opportunities for public comment and feedback on scientific advances.
While such opportunities may allow scientists to gain recognition and understanding for their work among non-specialists, they also have the potential to fuel inaccurate public perceptions about the current state of scientific progress, potential for application, and associated risks and uncertainties (Kamenova and Caulfield, 2015). Scientists, clinicians, bioethicists, science communications professionals at academic and research institutions, and industry spokespersons should strive to ensure that benefits, risks, and uncertainties of stem cell science are not understated, misrepresented or overstated (see Recommendation 3.3.4.1). Additionally, due to public interest and concern in the ethics of human pluripotent stem cell research, and in order to ensure complete transparency of research and translational activities, the origin of stem cell materials should be clearly specified in all communications.
Care should be exercised throughout the science communication process, including in the promotion of research and translation activities, as well as in the presentation of scientific results, the use of social media, and in any communication with print and broadcast media. Particular caution should be exercised when preparing press releases and other types of promotional material. Researchers should make efforts to seek timely corrections of inaccurate or misleading public representations of research projects, achievements, or goals. Scientists should also be particularly careful about disclosing research findings that have not passed peer review, as premature reporting can undermine public confidence if findings are subsequently disproven. For example, if researchers post online preprints that have not been peer-reviewed, readers should be informed of the preliminary nature of such manuscripts.
Researchers must intentionally avoid and correct inaccurate misconceptions in any communications regarding chimeras, genome editing, and other issues with a long history in the public imagination. While organoids, chimeras, embryo models, and other stem cell-based models are useful research tools offering possibilities for further scientific progress, limitations on the current state of scientific knowledge and regulatory constraints must be clearly explained in any communications with the public or media. Suggestions that any of the current in vitro models can recapitulate an intact embryo, human sentience or integrated brain function are unfounded overstatements that should be avoided and contradicted with more precise characterizations of current understanding. This is particularly relevant to brain organoids and human-animal chimeras, where any statements implying human cognitive abilities, human consciousness or self-awareness, as well as phrases or graphical representations suggesting human-like cognitive abilities risks misleading the public and sowing doubts about the legitimate nature of such research. Likewise, forward-looking statements on inherently uncertain developments, such as predictions on time required until clinical application, the likelihood of product approval, or speculation on the potential economic impact of currently unrealized technologies, must be accurate, circumspect, and restrained.
The stem cell community should work closely with communications professionals at their institution to create information resources that are easy to understand without oversimplifying, and that do not underplay risks and uncertainties or exaggerate potential benefits. Similarly, research-sponsoring institutions and communications professionals have a responsibility to ensure that any informational materials referring to research achievements adhere to these principles. Additionally, the scientists in charge of the research findings that are featured in informational materials should review and agree to the content prior to release. For potentially sensitive or high-profile cases, it is advisable to seek additional comments from independent experts to ensure objectivity and balance, place research in context of existing body of evidence, and help identify study limitations and alternative interpretations of key findings.
Communications about Clinical Trials
Recommendation 4.2: When describing clinical trials in the media or in medical communications, investigators, sponsors, and institutions should provide balance and not emphasize statistically significant secondary results when pre-specified primary efficacy results are not statistically significant.
Too often, studies reporting statistically non-significant primary outcomes are “spun” by appealing to other findings, such as statistically significant secondary outcomes (Boutron et al., 2010). Such reporting practices can distort the medical and public interpretation of trial results. When communicating clinical research results, scientists, institutions, and journalists should clearly state the pre-specified primary endpoint of the study and whether or not it was reached with statistical significance. This standard should apply to conference abstracts, press releases directed at investors and other parties, and peer-reviewed publications.
Clinical trials designed to evaluate safety and efficacy should not be described using language that might suggest their primary intent to be the delivery of care, as this may lead to confusion about the risk/benefit ratio of study participation (see also Recommendation 3.4.2.1). Communications about ongoing studies should explain that clinical efficacy is not established, and that the results may reveal the intervention to be ineffective or, in some cases, harmful.
Scientists should collaborate with patient and advocacy groups promote a clear understanding of the clinical research process and the current state of progress in developing stem cell-based treatments for specific medical conditions. Accordingly, all involved in clinical research, including not only investigators and sponsoring institutions but also patients, study participants, funding bodies, families and advocacy groups, should exercise caution when communicating with the public. Additionally, researchers should exercise great care when making forward-looking statements regarding the potential outcome of any study.
Communications about Clinical Care
Recommendation 4.3: The provision of information to patients considering stem cell-based interventions must be consistent with the primacy of patient welfare, scientific and ethical integrity.
The provision of accurate information on risks, limitations, possible benefits, and available alternatives to patients is essential in the delivery of healthcare. Provision of clinical information, including recommendations on use, should center on the importance of consultation with medical professionals directly familiar with the individual patient’s case, and the seeking of independent expert opinion. The goal of clinical communications is to enable autonomous, well-informed decision-making by patients.
Language suggesting that novel interventions are “curative” should be avoided in the absence of evidence of complete or permanent relief of a disease or condition. A cure means that individuals do not experience ongoing morbidity or adverse effects from the disease or condition targeted by the intervention. Cures must be validated with long-term studies demonstrating that the treated patients have a similar annual death rate from all causes to that of the disease-free population group of the same sex and age distribution (Easson et al., 1963; Frei et al., 1971; Ravi et al., 2018).
Given the novelty of stem cell-based interventions and the fact that many countries do not have well-established regulatory pathways governing the introduction of novel medical products into clinical use, clinicians should exercise restraint in their communications regarding the clinical utility of such treatments. The use of patient anecdotes, testimonials or other language that could be construed as promotional, promissory, or suggestive of clinical effectiveness in reference to stem cell-based interventions for which efficacy has not been established is to be avoided. In the event that new stem cell-based interventions are authorized for use for a specified indication, care must be taken to avoid communications that might indicate or suggest to patients that such intervention is efficacious for other indications.
Regulatory and law enforcement authorities are encouraged to investigate and, when appropriate, restrict unsupported marketing claims made by commercial actors, to the extent that these violate relevant consumer protection, truth in advertising, securities, and commerce laws within a given jurisdiction.
When approved stem cell products are used for off-label indications, communications should clearly specify that such interventions will be used on an off-label basis. Such communications should explain the difference between administering products according to the marketing labels approved by regulatory bodies and off-label use that lacks such approval. Many countries have legal restrictions concerning marketing claims related to off-label use. Such constraints are intended to ensure that advertising claims are evidence-based and promotional rhetoric does not go beyond credible safety and efficacy data and related regulatory approvals.
Return to TOC | 2021 Guidelines for Stem Cell Research and Clinical Translation
5. Standards in Stem Cell Research
Translation of cell-based interventions is a collaborative endeavor among scientists, clinics, industry, regulators, and patients. Standards help enable such collaborations and support efficient clinical translation in many ways.
Return to TOC | 2021 Guidelines for Stem Cell Research and Clinical Translation
Translation of cell-based interventions is a collaborative endeavor among scientists, clinics, industry, regulators, and patients. Standards help enable such collaborations and support efficient clinical translation in many ways. For instance, they allow scientists to compare the outcomes of trials and enable clinics to reproduce treatments reported in published studies. Regulatory standards also reduce the costs of uncertainty for private actors, facilitate an independent review, and engender trust among patients.
Standards Development
Recommendation 5.1: Researchers, industry, and regulators should work towards developing and implementing standards on design, conduct, interpretation, preclinical safety testing, and reporting of research in stem cell science and medicine.
There are numerous areas where standards development would greatly advance the science of stem cells and its clinical application. Gaps and priorities for standard developments should be extensively and thoroughly studied to meet the rapid advancement of stem cell science and medicine. Particular opportunities topics include but not limited to standards for:
Source materials: (a) consent, (b) procurement, (c) manufacturing regulations, (d) cell potency assays primary quality attributes, (e) reference materials for calibrating instruments;
Process controls: (a) detection and inspection, (b) biobanking of stem cells, (c) minimally acceptable changes during cell culture, (d) method of delivery and selection of recipients for novel stem cell-based interventions, (e) reporting of animal experiments, (f) design of trials, (g) reporting of trials, (h) principles for defining information in datasets as “sensitive” such that there is a justified withholding or delay of study reporting.
Instrument, facility, environment and personnel;
Analytical methods; and
Data processing.
Scientists, regulators, funders, patient groups, and others involved in stem cell research should collaborate on the timely development of standards for stem cell research and translation. To promote common and universal standards for consent and procurement of biomaterials, the ISSCR has provided template donor consent forms (Appendix 2).
Revisiting the ISSCR Guidelines
Recommendation 5.2: The ISSCR guidelines should be periodically revised to accommodate scientific advances, new challenges, and evolving social priorities.
New scientific opportunities and ethical challenges in the conduct of stem cell research and stem cell-based interventions that are on the horizon must be addressed in a timely manner to ensure that science and medical care proceed in a socially responsible and ethically acceptable fashion. Periodic revision enhances the likelihood that the international scientific research community will be bound together by a common set of principles governing the performance of stem cell research.
Return to TOC | 2021 Guidelines for Stem Cell Research and Clinical Translation
Acknowledgements
Return to TOC | 2021 Guidelines for Stem Cell Research and Clinical Translation
These guidelines were prepared by the Task Force to Update the ISSCR Guidelines, charged with revising and updating ISSCR Guidelines for Stem Cell Research and Clinical Translation.
The task force thanks the many individuals and organizations who reviewed the draft guidelines and provided comments or otherwise contributed to our deliberations.
ISSCR Guidelines Update Task Force
Return to TOC | 2021 Guidelines for Stem Cell Research and Clinical Translation
Steering Committee
Chair - Robin Lovell-Badge, CBE, FRS FMedSci, Francis Crick Institute, UK
Melissa Carpenter, PhD, ElevateBio, USA
R. Alta Charo, J.D., University of Wisconsin, USA
Amander Clark, PhD, University of California, Los Angeles, USA
George Q. Daley, MD, PhD, Harvard Medical School, USA
Insoo Hyun, PhD, Harvard Medical School, USA
Jürgen Knoblich, PhD, IMBA-Institute of Molecular Biotechnology, Austria
Heather Rooke, PhD, Broad Institute, USA
Janet Rossant, CC, FRS, FRSC, Gairdner Foundation/SickKids, Canada
Douglas Sipp, RIKEN Center for Developmental Biology, Japan and Keio University School of Medicine, Japan
Working Group Members
Roger Barker, Cambridge Center for Brain Repair, UK
Tania Bubela, Simon Fraser University, Canada
Ali H. Brivanlou, The Rockefeller University, USA
Ellen Clayton, Vanderbilt University, USA
Yali Cong, Peking University, China
Jianping Fu, University of Michigan, USA
Misao Fujita, Kyoto University, Japan
Andy Greenfield, MRC Harwell Institute, UK
Steve Goldman, University of Rochester Medical Center, USA
Lori Hill, MD Anderson, USA
Rosario Isasi, University of Miami, USA
Jeffrey Kahn, Johns Hopkins University, USA
Kazuto Kato, Osaka University, Japan
Jin-Soo Kim, Seoul National University, Korea
Jonathan Kimmelman, McGill University, Canada
Debra Mathews, Johns Hopkins University, USA
Nuria Montserrat, Institute for Bioengineering of Catalonia (IBEC), Spain
Megan Munsie, University of Melbourne, Australia
Hiromitsu Nakauchi, Stanford University, USA/University of Tokyo, Japan
Luigi Naldini, Università Vita-Salute San Raffaele, Italy
Gail Naughton, Histogen, USA
Kathy Niakan, Francis Crick Institute, UK
Ubaka Ogbogu, University of Alberta, Canada
Roger Pedersen, Stanford University, USA
Nicolas Rivron, IMBA-Institute of Molecular Biotechnology, Austria
Jeff Round, Institute of Health Economics, Canada
Mitinori Saitou, Kyoto University, Japan
Julie Steffann, Université Paris Descartes, France
Jeremy Sugarman, Johns Hopkins University, USA
Azim Surani, University of Cambridge, UK
Jun Takahashi, Kyoto University, Japan
Fuchou Tang, Peking University, China
Leigh Turner, University of Minnesota, USA
Patti Zettler, Ohio State University, USA
Xiaomei Zhai, Peking Union Medical College, China
Supported by ISSCR staff
Jack Mosher, Scientific Affairs, Senior Manager
Glori Rosenson, Director of Outreach
Return to TOC | 2021 Guidelines for Stem Cell Research and Clinical Translation
Appendices
Return to TOC | 2021 Guidelines for Stem Cell Research and Clinical Translation
Appendix 1. The Transfer of Human Stem Cells or their Direct Derivatives into Animal Hosts
-
Recommendation A6.1: Research involving the transfer of human stem cells or their direct neural and/or glial derivatives into the central nervous systems of postnatal animal hosts requires review by institutional animal research oversight committees supplemented by reviewer expertise in stem cell or developmental biology.
For protocols involving the transfer of human stem cells or their direct neural and/or glial derivatives such that they contribute to the central nervous systems of postnatal animal hosts, research review should be conducted by animal research review committees supplemented with expertise in stem cell or developmental biology. Review should be based on a reasonable extension of existing animal research standards, which are themselves based on rational, practical, fact-based assessments of the effects of research manipulations on cognitive and functional outcome measures, as well as on animal health and welfare.
Additional data collection and monitoring by animal research committees should be commensurate with the anticipated characteristics of the modified animal in the context of the proposed research. Issues regarding the possible change in or enhancement of an animal’s behavior or operationally-assessed cognition should be addressed through diligent application of accepted principles for the humane treatment and protection of animals in research, and primarily through regular animal research oversight mechanisms.
Monitoring and data collection should be based upon a sound assessment of the developmental trajectories of the animal host that may be further affected by taking into account the environmental and epigenetic context in which the donor genes or cells are going to be deployed. It should be grounded in existing knowledge of such trajectories, with reasonable scientific inferences as to their phenotypic and fate potential, with thorough reference to the physiological and behavioral tests and assessments currently available by which to assess the host species.
Research involving the modification of the central nervous system, as established with the introduction of human stem cells or their neural and/or glial derivatives in a way that they contribute to the brains or spinal cords of animal hosts, may attempt to model or directly mimic aspects of human neurological and neuropsychiatric function. As such, this research may demand specialized cognitive and behavioral assessments of the sort conducted in neuroscientific research. There may be an irreducible degree of uncertainty about the internal cognitive processes of any new animal model, in particular how it would manifest distress, anxiety, or other aspects of animal welfare. In such cases, as with transgenic animals, researchers and institutions should familiarize themselves with available options for behavioral response assessment. A baseline of normal behavioral data for the test species and strain should be available before experimentation is permitted, so as to enable clear and rapid identification of behavioral differences or abnormalities associated with treatment and/or human cell transfer. Investigators and institutions should also consider requiring limited pilot studies to produce initial data on the effects of experimental interventions on modified animals, monitoring all deviations from normal behaviors, with prescribed discussion with pertinent animal welfare committees before proceeding to definitive experiments.
Investigators and institutions should also make appropriate adjustments to research protocols to take into account new data or unanticipated responses from animal subjects that may inform or alter the continued permissibility of the animal’s participation in the study. These include identifying any novel signals suggesting a material change in an animal’s condition, comfort, or behavioral state or repertoire, whether by way of deterioration or enhancement. Regular reassessment of animal welfare during the course of experimentation is essential.
Research with a known, intended, or well-grounded significant potential to create some aspect suggestive of human cognition, self-awareness, behavior or behavioral pathology, while not prohibited, should be subject to close scrutiny, taking care to ensure the humane protection of animal subjects. Such studies require a clear and compelling justification, grounded in the potential for significant scientific breakthrough, clinical advance, or both.
Through retained advisors or committee diversity, animal research review committees should ensure that they have sufficient scientific and clinical expertise to make appropriate judgments concerning the matters discussed in these recommendations.
-
Recommendation A6.2: Researchers using large, complex animal models, such as livestock and non-human primates, should follow international standards for livestock animal and non-human primate research, which call for frequent monitoring of animals whenever there is the potential for unexpected outcomes and unanticipated phenotypes.
Best practices dictate that research with non-human primates (NHPs) should account for the following (Tardif et al. 2013):
Investigators must justify their choice of an NHP species in light of the goals of the study.
For some NHP species, temporary removal of an individual from its social group may cause it acute stress, and permanent removal may cause distress (the inability to cope with stress). Because of this variability, investigators and veterinarian staff must be aware of normal behaviors of individual NHPs and must know how to identify potential signs of stress and distress.
Because NHPs are valuable experimental subjects, they are often used in serial studies. Both the number of procedures and their consequent levels of burden must be strongly justified by investigators and monitored by trained veterinary staff.
Housing NHPs in social groups best replicates the social interactions they experience in the wild and thereby promotes species-typical behaviors and psychological well-being. For this reason, any singly housed modified NHPs should kept so for the minimum length of time required. The need for single housing should be reviewed by animal research committee members and veterinary staff. Because NHPs are social animals, single housing can produce a reduced range of species-typical behavior, increased environmental stressors, and self-inflicted wounding or withdrawn behavior. Not only could these outcomes affect the welfare of modified NHPs, but they might also confound investigators’ judgements about any potential behavioral changes caused by the transfer per se of human stem cells or their direct derivatives.
Best practices dictate that research with livestock animals should abide by the following standards:
Guide for the Care and Use of Laboratory Animals – guidance document required for PHS funded studies in US and for AAALAC accredited facilities worldwide https://www.aaalac.org/the-guide/
European standards, also a core reference resource for AAALAC https://www.aaalac.org/pub/?id=E900C0A9-EEB3-1C2E-6A3C-0C84FF348CDD
Research with Agricultural Animals and Wildlife. ILAR Journal, Volume 60, Issue 1, 2019, Pages 66–73. https://academic.oup.com/ilarjournal/article/60/1/66/5490285
Agricultural Animals as Biomedical Models: Occupational Health and Safety Considerations. ILAR Journal, Volume 59, Issue 2, 2018, Pages 161–167 https://academic.oup.com/ilarjournal/article/59/2/161/5196514#140647793
Appendix 2. Sample Informed Consent Documents for Procurement of Human Biomaterials for Stem Cell Research
Appendix 3. Informed Consent Considerations for Procurement of Cells and Tissues for Stem Cell Research and Translation
-
The informed consent process for the procurement of cells and tissues for stem cell research and translation should cover the following statements, adapted to the particular project:
That the cells and tissues may be used in the derivation of continuously growing cell cultures, including production of embryonic or pluripotent stem cell lines.
That the embryos or tissues will be destroyed, or isolated cells altered, during the process of deriving totipotent or pluripotent cells for research.
That derived cells and/or cell lines might be deposited and stored in a repository many years and used internationally for future studies, many of which may not be anticipated at this time.
That cells and/or cell lines might be used in research involving genetic alteration of the cells, the generation of organoids (small organ models) or animal research (resulting from the transfer of human stem cells or their direct derivatives into animal models, or the introduction of human stem cells into animal embryos).
That the donation is made without any restriction or direction regarding who may be the recipient of transplants of the cells derived, except in the case of autologous transplantation or directed altruistic donation.
Whether the donation is limited to specific research purposes or is for broadly stated purposes, including research and/or clinical application not presently anticipated, in which case the consent shall notify donors, if applicable under governing law, of the possibility that permission for broader uses may later be granted and consent waived under appropriate circumstances by a human subjects review committee. The consent process should explore and document whether donors have objections to the specific forms of research and/or clinical application outlined in the research protocol.
Whether the donor may be approached in the future to seek additional consent for new uses or to request additional materials (such as blood or other clinical samples) or information.
Disclosure of what donor medical or other information and what donor identifiers will be retained, specific steps taken to protect donor privacy and the confidentiality of retained information, and whether the identity of the donor will be readily ascertainable to those who derive or work with the resulting stem cell lines, or any other entity or person, including specifically any oversight bodies and government agencies.
Disclosure of the possibility that any resulting cells or cell lines may have commercial potential, and whether the donor will or will not receive financial benefits from any future commercial development.
Disclosure of any present or potential future financial benefits to the investigator and the institution related to or arising from proposed research.
That the research is not intended to provide direct medical benefit to anyone including the donor, except in the sense that research advances may benefit the community.
That neither consenting nor refusing to donate biomaterials for research will affect the quality of care provided to potential donors.
That there are alternatives to donating human biomaterials for research, and an explanation of what these alternatives are.
For donation or creation of embryos for research, that the embryos will not be used to attempt to produce a pregnancy.
For donation of gametes, that they will not be used to create embryos unless explicit consent is obtained and the resulting embryos will not be used for reproductive purposes.
For experiments in embryonic stem cell derivation, somatic cell nuclear transfer, somatic cell reprogramming, parthenogenesis, or androgenesis, that the resulting cells or stem cell lines derived would carry some or all of the DNA of the donor and therefore be partially or completely genetically matched to the donor.
That nucleic acid sequencing of the resulting stem cell line is likely to be performed and this data may be stored in databases available to the public or to qualified researchers with confidentiality provisions, and that this may compromise the capacity for donation to remain anonymous and/or de-identified.
That the donor and/or biomaterials will be screened for infectious and possibly genetic diseases or markers of disease.
Whether there is a plan to share with the biomaterials donor any clinically relevant health information discovered incidentally during the course of research, and if so, what those plans are, including the right not to receive such results.
Appendix 4. Sample Material Transfer Agreement Document
-
Download the ISSCR SAMPLE FORM Microsoft Word document: Uniform Human Embryonic Stem Cell-related Materials Transfer Agreement
Appendix 5. Considerations for Genome Editing Research
-
Assessing tumorigenicity of genome-modified cell interventions
For gene-modified cell products early readouts of a potential tumorigenic risk could include the expansion of one or few dominant clones from a starting polyclonal graft in a (xeno)-transplanted host. The emergence of such dominant clones may highlight the occurrence within the administered cell population of some genotoxic events consequent to the genetic modification, such as integration of a gene transfer vector or editing-induced translocation nearby an oncogene. These random and presumably rare events may activate the tumorigenic potential of the oncogene and endow the affected cell with a gain-of-function mutation promoting its growth. It should be realized that cells carrying genotoxic events leading to a gain of function may not progress to the formation of a full-blown tumor in preclinical models for lack of proper supporting conditions, sufficient follow-up time or the small scale of the study. On the other hand, such could develop in humans, where more cells are administered and clinical persistence may extend far longer than in conventional preclinical models. Clonal tracking of administered cells in vivo has been primarily developed and validated as a safety readout in the field of hematopoietic stem cell gene therapy, where semirandom genome-wide insertions of vector provide a unique clonal marker of transduced cells. In studies using early generation retroviral vectors, expansion of rare clones carrying vector integration nearby certain oncogenes was often reported, both in animal models as well as in human subjects, and some of these clones eventually progressed to overt leukemia. In these cases, identification of a vector insertion next to an oncogene in the leukemic clone allows tracing the origin of the leukemia to the original genetic modification. Such clonal markers of gene-modified cells may not be available when using other engineering platforms such as genome editing or when the cell product does not undergo genetic engineering. Clonal tracking could still be attempted using surrogate readouts such as randomly acquired genomic mutations and monitoring the graft for the skewing from polyclonal to oligoclonal composition and the potential emergence and expansion of clones having a growth advantage, which may eventually progress to tumors.
Preclinical Safety and Efficacy Involving Genome Modified Cell Interventions
The following must be addressed and minimized through preclinical studies before initiating a first-in-human clinical trial.
Issues Particular to Gene Replacement
Semi-random insertion of exogenous DNA may cause genotoxicity when a sporadic insertion takes place near an oncogene and causes its activation by truncation and/or transcriptional activation or disrupts tumor suppressor genes. These events may be rare but due to the very large number of insertions typically occurring in some cell therapies, they may well occur within a cell product. The few cells bearing such insertions might then expand and become dominant in vivo because of the enhanced growth potential afforded by the mutation. Genome insertions are expected with integrating vector platforms (such as retro-/lenti-viral vectors or transposons) but may also occur inadvertently and to lower extent when episomal DNA (i.e. from AAV vectors or plasmids) become incorporated by non-homologous end joining (NHEJ) at sites of DNA double-stranded breaks (DSB). For integrating vectors, a design should be used that minimizes the risk of genotoxic insertions (i.e. reducing the extent of transcriptional transactivation or readthrough from insertion site). Some knowledge should also be acquired on the genome-wide insertion pattern in the selected cell type and any existing specific biases that may increase the risk of genotoxic insertions. The genomic distribution of vector insertion should be assessed by preclinical studies in the cultured treated cells as well after in vivo administration into recipients, which should be monitored for the emergence of dominant clones with genotoxic insertions. Information available from prior studies performed with the same or similar vector backbone and target cells might alleviate the requirement for new extensive investigation. For non-integrating platforms, the residual extent of insertion or lack thereof should be investigated or previously known.
The potential mobilization of the vector, whether integrated or maintained as an episome, upon superinfection of the engineered cells in the recipient by wild-type virus, and the possibility of recombination of the vector genome with the wild-type viral genome should also be considered among the potential long-term risk. It is expected that recombination of vector sequence with the parental viral genome would most often result in a replication-defective virus. However, the potential risk of incorporating a new and biohazardous gene in the viral gene pool should be considered and, if present, alleviated by adopting conditions minimizing such risk. Many integrating vectors derived from retro-/lenti-viruses are commonly designed to be “self-inactivating”. This design means that upon integration the viral long terminal repeats are deleted of most transcription activating sequences. Such deletion makes the rescue of proviral expression, and its capture by the superinfecting virus, highly unlikely.
Cytoplasmic and nuclear exposure to exogenous nucleic acids, whether of viral, plasmid or other origin, and their replication intermediates might activate the innate immune sensing machinery in the treated cells. This activation may in turn trigger detrimental and inflammatory responses, potentially spreading to neighboring cells. Such responses might be minimal and only have subtle effects. However, if their activation is more robust or sustained they might impact the ability to engraft and adversely affect the clonal composition and long-term stability of an engineered cell graft. Importantly, these responses might be substantially augmented by excess impurities, such as DNA fragments and residual plasmid in the final cell product. Thus, efforts should be made to reduce impurities in the vector preparation.
Pre-existing immunity to viruses used to make gene transfer vectors may limit their application in vivo. This might be due to the presence of high-titer neutralizing antibodies in the plasma that may inactivate the vector and thus block gene transfer. Another possibility is the recognition of residual viral components in the transduced cells by T-cells, which may lead to the immune-mediated clearance of the transduced cells. The latter response might also affect ex vivo engineered cells if administered shortly after vector exposure. These immune responses may impact the in vivo survival of engineered cells and should be appropriately investigated before clinical testing.
Issues Particular to Genome Editing
The first and best developed approach to genome editing exploits engineered endonucleases to deliver a DNA double-stranded break (DSB) to the intended target sequence. One main safety concern is the off-target activity of the nuclease. Extensive preclinical testing should be performed to interrogate the genome-wide specificity of the editing reagents using orthogonal techniques. The target sequence is first chosen to be uniquely represented in the genome and with limited or no occurrence of any highly similar homologous sequences bearing only a few mismatches. Bioinformatic prediction of potential off targets is then performed to rule out potential activity in known sensitive genomic sites (such as tumor suppressor genes). An experimental assessment of specificity is then performed on DNA in vitro or in cell lines exposed to high concentration of the nuclease by one or more techniques, thus generating a list of candidate off-target sites, which are also analyzed comparatively with the bioinformatic predictions. Finally, the top ranked off-target sites are interrogated by deep sequencing for targeting by the nuclease in the selected target cells in conditions best representative of the intended clinical protocol. These studies should be conducted with proper positive and negative controls to determine sensitivity thresholds. Standard or threshold acceptance values for off targets are hard to provide across platforms, target cells and applications, and should be determined accordingly to the intended use.
Large genomic alterations, deletions and translocations are also induced, albeit to lower extent than NHEJ and HDR-mediated repair, at the DNA DSB sites, and are all difficult to evaluate. This is particularly true for allele drop-outs due to large deletions, which can encompass large segments of DNA. These events may be of particular concern if they lead to hemizygosity or even homozygosity for a loss-of-function mutation in a tumor suppressor gene. The possible contribution to loss of heterozygosity by gene conversion in the course of repair of a DNA DSB should also be considered. Efforts should go into ruling out the occurrence of unwanted on-target genomic alterations above a threshold limit of detection and/or expectation. Moreover, the possible occurrence of genomic rearrangement involving sensitive loci should be cause for discarding the candidate reagents. When addressing the overall safety of a cell product that may comprise a small fraction of cells bearing genomic alterations below the threshold of detection one may be able to draw upon available past experience with gene- and cell-based interventions using the same or other platforms with the same target cells.
Biodistribution studies of genome edited cells in suitable xenogeneic immunocompromised recipients should be performed to establish comparable behavior to untreated cells. Targeted editing by nucleases may leave a genetic scar. Such scars may be traceable, depending on the mechanism of repair of the DNA DSB. NHEJ-mediated DSB repair usually introduces small nucleotide insertion/deletions (indels) at the target site, which can be identified by deep sequencing the locus. However, some editing events might be missed because the original sequence is reconstituted or has been lost by a large deletion or because it was involved in a translocation. If only one base has been changed, it will be difficult to distinguish it from a sequencing error. Homology directed repair (HDR) of DSB can more easily be tracked because of the templated sequence changes in the target locus. Whenever feasible, strategies should be adopted to allow reliable tracking of the edited cells, for instance by recoding part of the target sequence in the template to introduce a traceable genetic marker. These base changes might also serve to protect the template from the action of the nuclease and improve the efficiency of editing. The genetic modifications introduced during editing could be used to track the fate, survival and biodistribution of the edited cells and their progeny. These studies will help to establish safety and efficacy of the treatment and address the possible relationship of eventual adverse events with the editing process (i.e. to distinguish the possible origin of abnormal differentiation, growth or transformation of some edited cells vs. background disease or age-related events). However, some edited cells may still escape tracking. Tracking of cells edited by base editors or epigenetic editors (see below) may prove even more difficult or perhaps impossible.
DNA DSB might induce DNA damage response in a dose-dependent manner as well as other signaling and transcriptional responses in cells treated for editing. P53-mediated responses have the potential to induce cell senescence with long-term detrimental effects and selection of p53+/- or -/- variants. The occurrence, extent and specific modes of such responses to genome editing still need to be investigated in most target cells and applications. Furthermore, combination of DNA DSB with vectors used to deliver the repair template for HDR may induce cumulative activation of innate immune sensors and trigger more detrimental responses. Such responses might only have transient effects but if robust and prolonged they might impact the cell survival, extent and time to engraftment, clonal composition and long-term stability of an engineered cell or tissue graft.
There are continuously emerging new technology platforms which introduce new editing modalities with broader reach and potentially improved precision and safety. For example: Break-less editors, Base editors, Prime editors (Anzalone et al., 2020). These new strategies are expected to provide improved editing precision at the target site by diminishing the spectrum of potential outcomes and to alleviate the burden imposed on the target cell by the DNA DSB. However, these new strategies also raise specific issues concerning monitoring for off-target effects. Specific tests might need to be designed to address genome wide specificity of these editors. In particular, many of these editors exploit the editing domain of an enzyme with constitutive activity independent of the binding of the fusion protein to DNA. Thus, off-target activity might be displayed semi-randomly in the genome and thus independently from the nearby occurrence of homology to the intended target sequence. Because of its semi-random occurrence such off-target activity may escape detection when investigating bulk treated cells, where semi-randomly distributed rare events would become noise. A possible strategy to address this issue is to compare SNVs among several single cell-derived clones from the treated cells.
In vivo genome editing still remains challenging because it requires an effective and safe delivery of the editing machinery to sufficient numbers of the relevant cell type. Current platforms support either stable high-level expression of editors with concomitant increased risk of toxicity, off-target activity and immunogenicity (such as when using AAV vectors) or they fail to achieve satisfactory efficacy due to low expression level. Nanoparticle based delivery methods represent a promising approach for short-term expression, but are still difficult to target to tissues other than the liver. Furthermore, most genome editors comprise at least some components of bacterial origin and are thus likely to be immunogenic. The sustained expression or even the residual presence of such material in the edited cells might impact their survival in vivo and this risk should be appropriately investigated before clinical testing.
Appendix 6. Informed Consent Standard for Stem Cell-Based Interventions Offered Outside of Formal Clinical Trials
-
Download a PDF of ISSCR Informed Consent Standards for Stem Cell-Based Interventions, Version 1.0, 12 August 2019
Return to TOC | 2021 Guidelines for Stem Cell Research and Clinical Translation
Glossary
Return to TOC | 2021 Guidelines for Stem Cell Research and Clinical Translation
Definitions and discussion of terminology relevant to these guidelines is found in Glossary pages G.1 – G.5. by using the left navigation or the Next button. Other definitions can be found at http://stemcells.nih.gov.
-
Blastocyst: The stage of preimplantation embryo development that, for humans, occurs around day 5–6 after fertilization or intracytoplasmic sperm injection. The blastocyst contains a fluid filled central cavity (blastocoele), an outer layer of cells (trophectoderm) and an inner cell mass (ICM). The trophectoderm cells attach the embryo to the uterine wall, and the ICM forms the embryo proper. The human blastocyst hatches from the zona pellucida (a surrounding glycoprotein shell) around days six-seven after fertilization. Thereafter, and coupled to implantation, the ICM of the blastocyst begins to organize itself into a flattened embryonic disc and associated amnion.
Cleavage stage embryo (preimplantation stage): The embryonic stage that follows the first division of the zygote and ends upon morula compaction; precise stages include the two-cell, four-cell, eight-cell and 16-cell embryo. In humans, each cleavage division takes around 18-24 hours.
Embryo: The term “embryo” has been defined and used differently in various biological contexts as discussed below.
In this document, the term “embryo” is used generically to describe all stages of development from the first cleavage of the fertilized ovum to nine weeks post fertilization in the human, including the placenta and other extraembryonic membranes.
More precise terms have been used to describe specific stages of embryogenesis; for example, the two, four and eight cell stages, the compacting morula and the blastocyst all describe particular stages of preimplantation embryonic development.
Prior to implantation, the embryo represents a simple cellular structure with minimal cellular specialization, but soon after implantation a defined structure called the primitive streak begins to form and marks the future posterior region of the embryo. After this time twinning of the embryo can no longer occur as there is irreversible commitment to the development of more complex and specialized tissues and organs.
Classical embryology used the term embryo to connote different stages of post-implantation development (for example, the primitive streak and onwards to fetal stages). Indeed, Dorland’s Illustrated Medical Dictionary (27th edition,1988 edition, W. B. Saunders Company) provides the definition “in animals, those derivatives of the fertilized ovum that eventually become the offspring, during their period of most rapid development, i.e., after the long axis appears until all major structures are represented. In man, the developing organism is an embryo from about two weeks after fertilization to the end of seventh or eighth week.” An entry in Random House Webster’s College Dictionary reads “in humans, the stage approximately from attachment of the fertilized ovum (egg or MII oocyte) to the uterine wall until about the eighth week of pregnancy.” However, the nomenclature is often extended by modern embryologists for the human to include the stages from first cleavage of the fertilized ovum onwards to seven to nine weeks post fertilization, after which the term fetus is used.
Fetus: In this document, the term “fetus” is used to describe post-embryonic stages of human prenatal development, after major structures have formed. In humans, this period is from eight to nine weeks after fertilization until birth. The term is often not used in animals where “embryo” is used for any stage from fertilization to term.
Stem cell-based embryo models: Advances in cellular engineering make possible the assembly, differentiation, aggregation, or re-association of cell populations in a manner that models or recapitulates key stages of embryonic development. Such experimental systems can provide essential insights into embryo and tissue development but raise concerns when such structures achieve complexity to the point where they might realistically manifest the ability to undergo further integrated development if cultured for additional time in vitro. There are two types of stem cell-based embryo models.
Non-integrated stem cell-based embryo models: These stem cell-based embryo models will experimentally recapitulate some, but not all aspects of the peri-implantation embryo, for example differentiation of the embryonic sac or embryonic disc in the absence of extraembryonic cells. These stem cell-based embryo models do not have any reasonable expectations of specifying additional cell types that would result in formation of an integrated embryo model. Gastruloids are an example of a non-integrated stem cell-based embryo model.
Integrated stem cell-based embryo models: These stem cell-based embryo models contain the relevant embryonic and extra-embryonic structures and could potentially achieve the complexity where they might realistically manifest the ability to undergo further integrated development if cultured for additional time in vitro. Integrated stem cell-based embryo models could be generated from a single source of cells, for example expanded potential human pluripotent stem cells capable of coordinately differentiating into embryonic and extraembryonic structures. Alternatively, integrated stem cell-based embryo models could also be generated through the formation of cellular aggregates where extraembryonic/embryonic cells from one source are combined with embryonic/extraembryonic cells from different sources to achieve integrated human development. This might include using non-human primate cells as one of the sources. Previous restrictions on preimplantation human embryo culture (the “14-day/primitive streak rule”) were not written to apply to integrated stem cell-based embryo models. Thus, these guidelines specify the imperative for specialized review when such research is designed to model the integrated development of the entire embryo including its extraembryonic membranes. A guiding principle of review should be that the integrated stem cell-based embryo models should be used to address a scientific question deemed highly meritorious by a rigorous review process. Blastoids are an example of an integrated stem cell model.
Morula: The compacting grape-like cluster of 16 cells, typically formed by the human embryo four days after fertilization.
Nuclear Transfer: This process involves the insertion of a nucleus of a cell into an ovum from which the nuclear material (chromosomes) has been removed. The ovum will reprogram (incompletely) the cell nucleus to begin development again. Embryos created by nuclear transfer are typically abnormal and often die during development, but rarely are capable of development to term. ICMs from blastocysts derived by nuclear transfer can form apparently normal embryonic stem cells.
Organoid: A tissue culture-derived structure growing in 3D and derived from stem cells that recapitulate the cell composition and a subset of the physiological functions of an organ through principles of self-organization.
Parthenogenetic embryo: Activation of the unfertilized mammalian ovum (usually accompanied by duplication of the haploid genome) can result in embryonic development, and embryonic stem cells can be derived from the ICMs of parthenogenetic blastocysts. After uterine transfer, parthenogenetic embryos of non-human animals have been observed to progress through early post-implantation development but further development is compromised by an underdeveloped placental system that prevents normal gestation. Gynogenesis is a particular form of parthenogenesis in which an embryo is created from the genetic contributions (female pronuclei) of two different zygotes. Androgenesis entails creation of an embryo that incorporates the male pronuclei from two different zygotes.
Zygote: The fertilized single cell pronuclear ovum (egg), typically observed in humans between 20-35 hours after insemination with sperm.
-
Pluripotent: The state of a single cell that is capable of differentiating into all tissues of an organism, with exception of the extraembryonic cell types.
Multipotent: The state of single cells that are capable of differentiating into multiple cell types, but not all of the cells of an organism. Multipotent cells, exemplified by the hematopoietic stem cell, give rise to a range of cells within a specific tissue. Within the developing organism multipotent cells may give rise to derivatives of more than one embryonic germ layer, as for mesendodermal progenitors. In the adult, multipotent cells are typically restricted to becoming derivatives of a specific germ layer (endoderm, ectoderm, mesoderm), organ, or tissue.
Teratoma: A benign, encapsulated mass of complex differentiated tissues comprising elements of all three embryonic germ layers: ectoderm, endoderm, and mesoderm. In the context of stem cell research, the teratoma assay entails injection of cell populations into immune-deficient murine hosts to assess their pluripotency (their capacity to produce derivatives from all three germ layers). These structures are distinct from teratocarcinomas in which, in addition to the differentiated derivatives, undifferentiated stem cells persist.
Totipotent: The state of a cell that is capable of giving rise to all types of differentiated cells found in an organism, as well as the supporting extra-embryonic structures of the placenta. A single totipotent cell might, by division in utero, reproduce the whole organism, but to date this has only been demonstrated by zygotes or blastomeres of early cleavage stage embryos.
Unipotent: The state of single cells that are capable of differentiating only along a specific cell lineage and are exemplified by lineage-committed progenitors of the hematopoietic system (for example, erythroblasts). Unipotent stem cells undergo self-renewal and differentiation along a single lineage, as exemplified by the spermatogonial stem cell.
-
Chimera: An organism carrying cell populations derived from two or more (genetically distinct) sources, where the latter include zygotes, later stage embryos, liveborn animals, or cells grown in culture. N.B. While rare, some humans are natural chimeras due to the aggregation of two preimplantation stage embryos. More commonly, cells may cross the placental barrier from mother to fetus or vice versa and persist in the ‘host’ for life (Madam 2020). The word chimera should, therefore, be used as a neutral scientific term, in contrast to its mythological origins.
Interspecies chimeras: Interspecies chimeras are animals containing integrated cellular contributions from another species. The degree of contribution can range from minor to extensive. For example, chimeras can be derived when human stem cells are transferred into non-human embryos. There are three types of true human-animal chimeras bearing special concern: (a) those that have the capacity for widespread chimerism, and (b) those that have a significant degree of chimerism to the central nervous system and (c) those that have chimerism of the germline. Human-to-non-human primate chimeras or cellular xenotransplants formed at any stage of development warrant particular attention. For additional guidance on the review of human-animal chimeras, please consult the ISSCR white paper on chimeras (Hyun et al., 2020).
Cellular transplants into postnatal animal hosts: Although formally the resulting animal can still be classed as chimera, where human cells with limited fate, in terms of cell type or tissue distribution are introduced into defined positions in postnatal animals (or late embryonic stages), this is usually referred to as a transplant or graft into a host. The graft can be homotopic, where it can integrate into the host tissue, or ectopic, where it may develop as a defined structure. Unless the methods involve transplanting human germ cells into animal gonads, these types of experiment will generally be of little concern, although they should be subject to review by an animal ethics committee.
-
Allogeneic transplantation: Refers to the transplantation of cells from a donor to another person, either related (as when from a sibling or parent) or from an unrelated individual. In hematopoietic stem cell transplantation, unrelated donors may be identified from large donor registries as being histocompatible or matched to the transplant recipient at a series of human leukocyte antigens known to mediate transplant rejection. Allogeneic hematopoietic stem cell transplantation carries with it the potential for the donor’s transplanted cells to mount an immune attack against the recipient (graft versus host disease), while solid organ transplant carries the risk of the recipient’s immune system rejecting the allograft. Both clinical settings require the use of immunosuppressive drugs, which in the case of solid organ transplant recipients must be taken lifelong, placing them at risk of infectious complications.
Autologous transplantation: Refers to the transplantation to an animal or human patient of his/her own cells. Because the cells are recognized by the patient’s immune system as “self,” no rejection or immune incompatibility is observed. Consequently, autologous transplantation of cells typically carries fewer risks than allogeneic transplantation. Generation of embryonic stem cells by somatic cell nuclear transfer or derivation of induced pluripotent stem cells by reprogramming offers a potential source of autologous cells for many different transplantation studies, offering the theoretical advantage of immune compatibility.
Homologous use: Refers to intended therapeutic use of cells within their native physiological context, for example, the transplantation of hematopoietic stem cells to regenerate the blood, or the use of adipose tissue to reconstruct a breast.
Non-homologous use: Refers to intended therapeutic use of cells outside their native physiological context, for example, the transplantation of hematopoietic cells or mesenchymal stromal cells into the heart or brain.
Tumorigenicity: The property of cells that describes their potential for forming tumors, or an abnormal growth of cells.
-
Assent: In the context of clinical research, assent means the participant agrees to take part. To give assent means that the participant is engaged in research decision-making in accordance with his or her capacities. Children and adolescents who are legal minors cannot give legally valid informed consent, but they may be able to give assent. Assent demands that the legal minor provide affirmative agreement to participate in research.
Clinical research: Any systematic research conducted with human subjects or groups of human subjects or on materials from humans, such as tissue samples.
Clinical trials: Any research study that prospectively assigns human subjects or groups of human subjects to one or more health-related interventions to evaluate the effects on health outcomes. Interventions include but are not restricted to drugs, cells and other biological products, surgical procedures, radiological procedures, diagnostics, devices, behavioral treatments, process-of-care changes, preventive care.
Compensation: Payment for research subjects’ non-financial burdens incurred during the course of their research participation, most commonly their time, effort, and inconvenience.
Correlative studies: Studies, typically occurring within clinical trials, that explore the cause and effects of an intervention on biological targets involved in a disease process or linkages among groups or different elements of a group.
Incidental finding: A discovery concerning an individual research participant or tissue donor that does not relate directly to the aims of a study but that has potential health or reproductive importance for the individual.
Minimal risk: Risk from procedures to human subjects or tissue donors that is comparable to the probability and the magnitude of harms that are ordinarily encountered in daily life or during the performance of routine physical or psychological examinations or tests.
Minor increase over minimal risk: An increment in risk that is only a fraction above the minimal risk threshold and considered acceptable by a reasonable person.
Observational studies: A type of clinical research where investigators observe human subjects or groups of human subjects to measure variables of interest; the assignment of subjects into a treated group versus a control group is not controlled by the investigator.
Reimbursement: Repayment for research subjects’ out-of-pocket expenses incurred during their participation in research.
Sham procedures: Procedures used as controls in clinical trials that mimic experimental procedures for research subjects in the “treatment” arm. These are performed to prevent research subjects and physicians assessing their outcomes and from knowing which arm of the trial the subject has been enrolled in. They are also sometimes performed to control for the effects that treatment delivery (rather than the treatment per se) has on a disease process. Sham procedures vary in their invasiveness. Examples include saline injections (where research subjects are injected with saline instead of cells), sham cardiac catheterization (where research subjects receive cardiac catheterization but are not injected with cells), and partial burr holes to the cranium (where researchers imitate the experience of receiving brain surgery by drilling a depression in the skull).
Undue inducement: An offer or reward so attractive that it threatens to impair the ability of prospective research subjects or donors to exercise proper judgment, or it encourages them to agree to procedures for which they are strongly averse.
Return to TOC | 2021 Guidelines for Stem Cell Research and Clinical Translation
References
Return to TOC | 2021 Guidelines for Stem Cell Research and Clinical Translation
Academy of Medical Sciences (2011). Animals containing human material. http://www.acmedsci.ac.uk/policy/policy-projects/animals-containing-human-material/
American College of Obstetricians and Gynecologists (2006). Using preimplantation embryos for research. ACOG Committee Opinion No. 347. Obstet. Gynecol. 108, 1305–1317.
Bacman, S., Williams, S., Pinto, M. et al. Specific elimination of mutant mitochondrial genomes in patient-derived cells by mitoTALENs. Nat Med 19, 1111–1113 (2013). https://doi.org/10.1038/nm.3261
Bacman SR, Kauppila JHK, Pereira CV, et al. MitoTALEN reduces mutant mtDNA load and restores tRNAAla levels in a mouse model of heteroplasmic mtDNA mutation [published correction appears in Nat Med. 2018 Oct 5;:]. Nat Med. 2018;24(11):1696–1700. doi:10.1038/s41591-018-0166-8
Benjaminy S, Kowal SP, MacDonald IM, Bubela T. Communicating the promise for ocular gene therapies: challenges and recommendations. Am J Ophthalmol. 2015 Sep;160(3):408-415.e2. doi: 10.1016/j.ajo.2015.05.026. Epub 2015 May 30. PMID: 26032192.
Boer, G. J. (1994). Ethical guidelines for the use of human embryonic or fetal tissue for experimental and clinical neurotransplantation and research. Journal of Neurology 242, 1–13.
Boutron, I., Dutton, S., Ravaud, P., and Altman, D.G. (2010). Reporting and interpretation of randomized controlled trials with statistically nonsignificant results for primary outcomes. JAMA 303, 2058-2064.
Bubela T, McCabe C, Archibald P, Atkins H, Bradshaw SE, Kefalas P, Mujoomdar M, Packer C, Piret J, Raxworthy M, Soares M, Viswanathan S. Bringing regenerative medicines to the clinic: the future for regulation and reimbursement. Regen Med. 2015;10(7):897-911. doi: 10.2217/rme.15.51. Epub 2015 Nov 13. PMID: 26565607.
Camacho, L.H., Bacik, J., Cheung, A., and Spriggs, D.R. (2005). Presentation and subsequent publication rates of phase I oncology clinical trials. Cancer 104, 1497-1504.
Costa-Borges, N., Nikitos, E., Spath, K. et al. (2020) First registered pilot trial to validate the safety and effectiveness of maternal spindle transfer to overcome infertility associated with poor oocyte quality. Fertility & Sterility (Abstract only) 114, issue 3, supplement , e71-e72 https://doi.org/10.1016/j.fertnstert.2020.08.220
Council for International Organizations of Medical Sciences (2016). International Ethical Guidelines for Health-related Research Involving Humans. https://cioms.ch/wp-content/uploads/2017/01/WEB-CIOMS-EthicalGuidelines.pdf
Department of Health, and Education and Welfare (1979). Report of the National Commission for the Protection of Human Subjects of Biomedical and Behavioral Research (The Belmont Report). 44 Fed. Reg. 23, 192.
ESHRE Taskforce on Ethics and Law (2001). The moral status of the pre-implantation embryo. Hum. Reprod. 17, 1409-1419.
Easson EC, Russell MH. Cure of Hodgkin’s disease. BMJ. 1963;5347:1704–7.
Ethics Committee of American Society for Reproductive Medicine (2013). Donating embryos for human embryonic stem cell (hESC) research: a committee opinion. Fertil. Steril. 100, 935-939.
European Parliament and Council of the European Union (2001). Directive 2001/20/EC of the European Parliament and of the Council of 4 April 2001 on the approximation of the laws, regulations and administrative provisions of the Member States relating to the implementation of good clinical practice in the conduct of clinical trials on medicinal products for human use. (Official Journal of the European Union).
European Science Foundation (2000). Good scientific practice in research and scholarship. Science Policy Briefing. http://www.esf.org/fileadmin/Public_documents/Publications/ESPB10.pdf
Fisher, M., Feuerstein, G., Howells, D.W., Hurn, P.D., Kent, T.A., Savitz, S.I., and Lo, E.H. (2009). Update of the stroke therapy academic industry roundtable preclinical recommendations. Stroke 40, 2244-2250.
Flory, J., and Emanuel, E. (2004). Interventions to improve research participants' understanding in informed consent for research: a systematic review. JAMA 292, 1593-1601.
Food and Drug Administration (2017). FDA Announces Comprehensive Regenerative Medicine Policy Framework. https://www.fda.gov/news-events/press-announcements/fda-announces-comprehensive-regenerative-medicine-policy-framework
Freeman, G.A., and Kimmelman, J. (2012). Publication and reporting conduct for pharmacodynamic analyses of tumor tissue in early-phase oncology trials. Clinical Cancer Research 18, 6478-6484.
Frei, E. 3rd & Gehan, E. A. Definition of cure for Hodgkin’s disease. Cancer Res. 31, 1828–1833 (1971).
Fung M, Yuan Y, Atkins H, Shi Q, Bubela T. Responsible Translation of Stem Cell Research: An Assessment of Clinical Trial Registration and Publications. Stem Cell Reports. 2017 May 9;8(5):1190-1201. doi: 10.1016/j.stemcr.2017.03.013. Epub 2017 Apr 13. PMID: 28416287; PMCID: PMC5425617.
Haimes, E., Skene, L., Ballantyne, A.J., Caulfield, T., Goldstein, L.S., Hyun, I., Kimmelman, J., Robert, J.S., Roxland, B.E., Scott, C.T., et al. (2013). Position statement on the provision and procurement of human eggs for stem cell research. Cell Stem Cell 12, 285-291.
Henderson, V.C., Kimmelman, J., Fergusson, D., Grimshaw, J.M., and Hackam, D.G. (2013). Threats to validity in the design and conduct of preclinical efficacy studies: a systematic review of guidelines for in vivo animal experiments. PLoS Medicine 10, e1001489.
Hudson, G., Takeda, Y. & Herbert, M. Reversion after replacement of mitochondrial DNA. Nature 574, E8–E11 (2019). https://doi.org/10.1038/s41586-019-1623-3
Human Fertilization and Embryology Authority (2016). Scientific review of the safety and efficacy of methods to avoid mitochondrial disease through assisted conception: 2016 update. https://www.hfea.gov.uk/media/2611/fourth_scientific_review_mitochondria_2016.pdf
Human Fertilization and Embryology Authority (2019). Code of Practice, 9th Edition. https://portal.hfea.gov.uk/media/1527/2019-12-16-code-of-practice-9th-edition-december-2019.pdf
Hyslop LA, Blakeley P, Craven L, Richardson J, Fogarty NM, Fragouli E, Lamb M, Wamaitha SE, Prathalingam N, Zhang Q, O’Keefe H. Towards clinical application of pronuclear transfer to prevent mitochondrial DNA disease. Nature. 2016 Jun 8 534: 383-386.
Hyun, I. (2013). Therapeutic hope, spiritual distress, and the problem of stem cell tourism. Cell Stem Cell12, 505-507.
Hyun, I., Taylor, P., Testa, G., Dickens, B., Jung, K. W., McNab, A., Roberston, J., Skene, L., and Zoloth, L. (2007). Ethical standards for human-to-animal chimera experiments in stem cell research. Cell Stem Cell 1, 159–163.
International Society for Stem Cell Research (ISSCR). (2006). Guidelines for the conduct of human embryonic stem cell research. http://www.isscr.org/docs/default-source/hesc-guidelines/isscrhescguidelines2006.pdf.
International Society for Stem Cell Research (ISSCR). (2008). Guidelines for the clinical translation of stem cells. http://www.isscr.org/docs/default-source/clin-trans-guidelines/isscrglclinicaltrans.pdf.
International Society for Stem Cell Research (ISSCR). (2016). Guidelines for Stem Cell Research and Clinical Translation. https://www.isscr.org/docs/default-source/all-isscr-guidelines/guidelines-2016/isscr-guidelines-for-stem-cell-research-and-clinical-translationd67119731dff6ddbb37cff0000940c19.pdf?sfvrsn=4
Madan K. Natural human chimeras: A review. Eur J Med Genet. 2020 Sep;63(9):103971. doi: 10.1016/j.ejmg.2020.103971. Epub 2020 Jun 18. PMID: 32565253.
National Academy of Sciences, National Academy of Engineering, and Institute of Medicine. 2009. On Being a Scientist: A Guide to Responsible Conduct in Research: Third Edition. Washington, DC: The National Academies Press. https://doi.org/10.17226/12192.
National Academy of Medicine, National Academy of Sciences, and the Royal Society. 2020. Heritable Human Genome Editing. Washington, DC.: The National Academies Press. doi: 10.17226/25665.
Institute of Medicine (2015). Sharing Clinical Trial Data: Maximizing Benefits, Minimizing Risks (Washington, DC: National Academies Press). doi:10.17226/18998.
Nuffield Council on Bioethics. 2016. Genome Editing: An Ethical Review. https://www.nuffieldbioethics.org/wp-content/uploads/Genome-editing-an-ethical-review.pdf
Institute of Medicine and National Research Council. 2010. Final Report of the National Academies' Human Embryonic Stem Cell Research Advisory Committee and 2010 Amendments to the National Academies' Guidelines for Human Embryonic Stem Cell Research. Washington, DC: The National Academies Press. https://doi.org/10.17226/12923.
Kamenova, K., and Caulfield, T. (2015). Stem cell hype: media portrayal of therapy translation. Science Translational Medicine 7, 278ps274.
Kang, E., Koski, A., Amato, P. et al. Reply to: Reversion after replacement of mitochondrial DNA. Nature 574, E12–E13 (2019). https://doi.org/10.1038/s41586-019-1624-2
Kang E, Wu J, Gutierrez NM, Koski A, Tippner-Hedges R, Agaronyan K, Platero-Luengo A, Martinez-Redondo P, Ma H, Lee Y, Hayama T, Van Dyken C, Wang X, Luo S, Ahmed R, Li Y, Ji D, Kayali R, Cinnioglu C, Olson S, Jensen J, Battaglia D, Lee D, Wu D, Huang T, Wolf DP, Temiakov D, Izpisua Belmonte JC, Amato P, Mitalipov S. Mitochondrial replacement in human oocytes carrying pathogenic mitochondrial DNA mutations. Nature 2016 DOI: 10.1038/nature20592
Kilkenny C, Browne WJ, Cuthill IC, Emerson M, and Altman DG (2010). Improving bioscience research reporting: the ARRIVE guidelines for reporting animal research. Plos Biol 8, e1000412.
Kimmelman, J., Mogil, J.S., Dirnagl, U. (2014). Distinguishing between Exploratory and Confirmatory Preclinical Research Will Improve Translation. PLoS Biol. 12, e1001863.
Kuriyan AE, Albini TA, Townsend JH, et al. Vision Loss after Intravitreal Injection of Autologous "Stem Cells" for AMD. N Engl J Med. 2017; 376(11):1047–1053. doi:10.1056/NEJMoa1609583
Landis, S.C., Amara, S.G., Asadullah, K., Austin, C.P., Blumenstein, R., Bradley, E.W., Crystal, R.G., Darnell, R.B., Ferrante, R.J., Fillit, H., et al. (2012). A call for transparent reporting to optimize the predictive value of preclinical research. Nature 490, 187-191.
Lau, D., Ogbogu, U., Taylor, B., Stafinski, T., Menon, D., and Caulfield, T. (2008). Stem cell clinics online: the direct-to-consumer portrayal of stem cell medicine. Cell Stem Cell 3, 591-594.
Mazur, P et al. 2019 “P-221 Mitochondrial Replacement Therapy Gives No Benefits to Patients of Advanced Maternal Age.” Paper presented to ASRM Scientific Conference and Expo, Philadelphia, Oct. 14-16. https://asrm.confex.com/asrm/2019/meetingapp.cgi/Paper/2347
Medical Professionalism Project (2002). Medical professionalism in the new millennium: a physician charter. Annals of Internal medicine 136, 243-246.
Munsie, M., and Hyun, I. (2014). A question of ethics: selling autologous stem cell therapies flaunts professional standards. Stem Cell Research 13, 647-653.
National Academies of Sciences, Engineering, and Medicine (2016). Mitochondrial Replacement Techniques: Ethical, Social, and Policy Considerations. (Washington, DC: The National Academies Press). doi:10.17226/21871.
National Institutes of Health (2014). Informed Consent Guidance for Human Gene Trials subject to the NIH Guidelines for Research Involving Recombinant or Synthetic Nucleic Acid Molecules (Office of Science Policy: Office of Biotechnology Activities). http://osp.od.nih.gov/sites/default/files/resources/IC2013.pdf
Nissanka N, Moraes CT. Mitochondrial DNA heteroplasmy in disease and targeted nuclease-based therapeutic approaches. EMBO Rep. 2020;21(3):e49612. doi:10.15252/embr.201949612
Nuremberg Code (1949). In Trials of War Criminals before the Nuremberg Military Tribunals under Control Council Law No 10, Vol 2 (Washington, DC: U.S. Government Printing Office), pp 181-182.
Office for Human Research Protections (OHRP) (1993). Research on Transplantation of Fetal Tissue SEC. 498A, National Institutes of Health Revitalization Act of 1993. http://www.hhs.gov/ohrp/policy/publiclaw103-43.htm.html
Percie du Sert N, Hurst V, Ahluwalia A, Alam S, Avey MT, Baker M, et al. (2020) The ARRIVE guidelines 2.0: Updated guidelines for reporting animal research. PLoS Biol 18(7): e3000410. https://doi.org/10.1371/journal.pbio.3000410
Ravi, P., Kumar, S.K., Cerhan, J.R. et al. Defining cure in multiple myeloma: a comparative study of outcomes of young individuals with myeloma and curable hematologic malignancies. Blood Cancer Journal 8, 26 (2018). https://doi.org/10.1038/s41408-018-0065-8:
Reddy, Pradeep et al. Selective Elimination of Mitochondrial Mutations in the Germline by Genome Editing. Cell, Volume 161, Issue 3 (2015) pp. 459 – 469. https://doi.org/10.1016/j.cell.2015.03.051
Saini, P., Loke, Y.K., Gamble, C., Altman, D.G., Williamson, P.R., and Kirkham, J.J. (2014). Selective reporting bias of harm outcomes within studies: findings from a cohort of systematic reviews. BMJ 349, g6501.
SB-180 Gene therapy kits: advisory notice and labels.(2019-2020). California State Legislature.
S.D. Tardif, K. Coleman, T.R. Hobbs, C. Lutz, “IACUC Review of Nonhuman Primate Research,” ILAR Journal 53, no. 2, 2013: DOI: 10.1093/ilar/ilt040.
Sena, E.S., van der Worp, H.B., Bath, P.M., Howells, D.W., and Macleod, M.R. (2010). Publication bias in reports of animal stroke studies leads to major overstatement of efficacy. PLoS Biol. 8, e1000344.
Sugarman J, Siegel A. How to determine whether existing human embryonic stem cell lines can be used ethically. Cell Stem Cell 2008; 3: 238-9. PMID: 18786411.
Sugarman J, Siegel AW. When embryonic stem cell lines fail to meet consent standards. Science 2008; 322: 379. PMID: 18927375.
Tsilidis, K.K., Panagiotou, O.A., Sena, E.S., Aretouli, E., Evangelou, E., Howells, D.W., Al-Shahi Salman. R., Macleod, M.R., Ioannidis, J.P. (2013). Evaluation of excess significance bias in animal studies of neurological diseases. PLoS Biol. 11, e1001609.
U.K. Department of Health (2014). Mitochondrial donation. Government response to the consultation on draft regulations to permit the use of new treatment techniques to prevent the transmission of a serious mitochondrial disease from mother to child. https://www.gov.uk/government/uploads/system/uploads/attachment_data/file/332881/Consultation_response.pdf
Wang, Tian et al. Polar Body Genome Transfer for Preventing the Transmission of Inherited Mitochondrial Diseases. Cell, 157 (2014), pp. 1591-1604. https://doi.org/10.1016/j.cell.2014.04.042
World Medical Association (2018). Declaration of Helsinki: Ethical principles for medical research involving human subjects. https://www.wma.net/policies-post/wma-declaration-of-helsinki-ethical-principles-for-medical-research-involving-human-subjects/
Wu, K., Zhong, C., Chen, T. et al. Polar bodies are efficient donors for reconstruction of human embryos for potential mitochondrial replacement therapy. Cell Res 27, 1069–1072 (2017). https://doi.org/10.1038/cr.2017.67
Yamada M, Emmanuele V, Sanchez-Quintero MJ, Sun B, Lallos G, Paull D, Zimmer M, Pagett S, Prosser RW, Sauer MV, Hirano M. Genetic Drift Can Compromise Mitochondrial Replacement by Nuclear Transfer in Human Oocytes. Cell stem cell. 2016 Jun 2;18(6):749-54.
Zhang, John et al. “Pregnancy derived from human zygote pronuclear transfer in a patient who had arrested embryos after IVF.” Reproductive biomedicine online vol. 33,4 (2016): 529-533. doi:10.1016/j.rbmo.2016.07.008
Return to TOC | 2021 Guidelines for Stem Cell Research and Clinical Translation
ISSCR's Guidelines for Stem Cell Research and Clinical Translation are strictly copyrighted by the society. No part of this document may be produced in any form without written permission of The International Society for Stem Cell Research. Contact isscr@isscr.org for more information.
©2022 by The International Society for Stem Cell Research. All rights reserved.
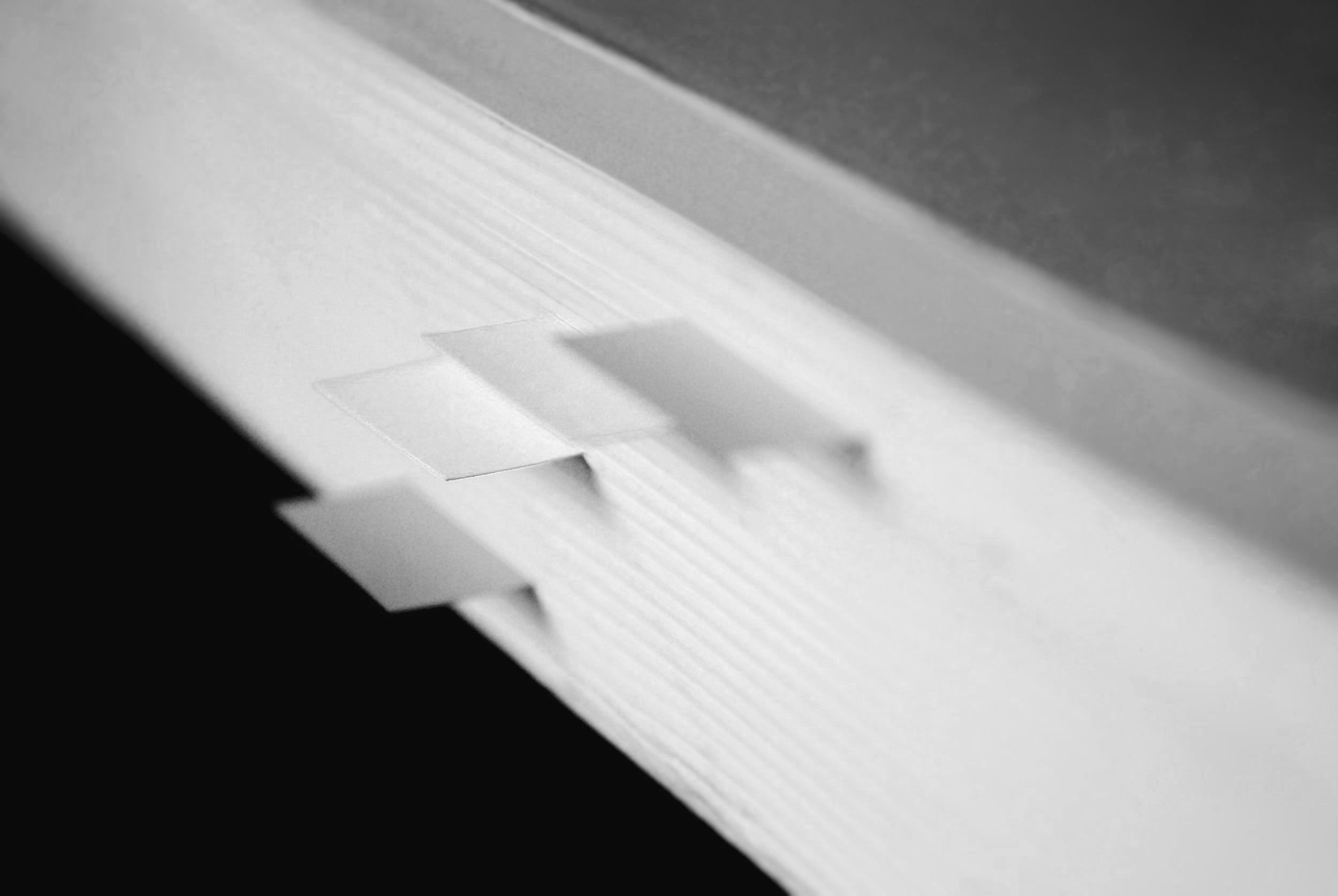
Key Topics
Excerpts from the 2021 guidelines update.
-
This page assembles the sections of the Guidelines that specifically address research involving the culture of human embryos. Other sections of the Guidelines may be relevant to such research (e.g., Section 2.1 Review Process, Section 2.3 Procurement and Informed Consent of Human Biological Materials, and Section 2.4 Derivation, Banking, and Distribution of Human Cell Lines).
2.2.2 Category 2
2.2.2 Category 2. Forms of research with embryos, certain chimeras, and stem cell-based embryo models that are permissible only after review and approval through a specialized scientific and ethics review process. A comprehensive review should be coordinated with other relevant oversight, such as that provided by human subjects review committees, in vitro fertilization (IVF) clinic oversight bodies, and animal research review processes (see 2.2.1), and the research should comply with local law and policy. All such research should have a compelling scientific rationale and necessitate the use of these materials rather than employ alternative models. The research should use the minimum number of embryos necessary to achieve the scientific objective. Forms of research requiring comprehensive review by a specialized review process encompass the following activities:
Procurement and use of IVF human embryos for research in vitro.
Procurement of human gametes to create research embryos in vitro.
Research that generates human gametes from any progenitor cell type in vitro, when this entails performing studies of fertilization that produce human zygotes and embryos. The gametes may be derived from human pluripotent stem cells, oogonia, or spermatogonial stem cells that have been maintained in vitro, and they may be genetically modified or not. Any human embryos obtained from such gametes must only be studied in vitro, or be used to derive stem cell lines, such as embryonic stem cells.
Research involving the genetic alteration of human embryos or gametes used to make embryos in vitro.
Derivation of new cell lines from human embryos (not confined to pluripotent cell lines).
Research involving the in vitro culture of human embryos where embryos are maintained in culture until the formation of the primitive streak or 14 days from fertilization, whichever occurs first.
Culture of Human Embryos Beyond formation of the primitive streak or 14 Days
It is currently not technically feasible to culture human embryos beyond formation of a primitive streak or 14 days post-fertilization. However, culture systems are evolving, making this a possibility in the near future. Understanding the primitive streak, early germ layer development and primordial germ cell formation in humans is crucial to improve our understanding of and interventions for infertility, in vitro fertilization, pregnancy loss, and developmental disorders that occur or originate soon after implantation. Research using embryos is also crucial to validate integrated stem cell-based embryo models, which in the future may provide a more practical alternative to understanding some aspects of early human development.
Recommendation 2.2.2.1: Given advancements in human embryo culture, and the potential for such research to yield beneficial knowledge that promotes human health and well-being, the ISSCR calls for national academies of science, academic societies, funders, and regulators to lead public conversations touching on the scientific significance as well as the societal and ethical issues raised by allowing such research. Should broad public support be achieved within a jurisdiction, and if local policies and regulations permit, a specialized scientific and ethical oversight process could weigh whether the scientific objectives necessitate and justify the time in culture beyond 14 days, ensuring that only a minimal number of embryos are used to achieve the research objectives.
-
This page assembles the sections of the Guidelines that specifically address research involving stem cell-based embryo models. Other sections of the Guidelines may be relevant to such research (e.g., Section 2.1 Review Process, Section 2.3 Procurement and Informed Consent of Human Biological Materials, and Section 4. Communications).
2.2.1 Category 1
2.2.1A Category 1A. Research determined to be exempt from a specialized scientific and ethics oversight process after being assessed by the appropriate existing mandates and committees for laboratory research. Category 1A research includes the following activities:
Research on stem cell culture systems that model specific stages of development or specific anatomic structures rather than the continuous development of an intact embryo or fetus. These would include but are not restricted to models of amnion formation, neural tube development, development of primordial germ cells, placental structures, 2D or 3D models of gastrulation or post-gastrulation events, and in vitro stem cell-derived organoids in culture that recapitulate most aspects of organ function, but not those that fall under subsequent categories.
2.2.1B: Category 1B. Research that is reportable to the entity or body responsible for the specialized scientific and ethics oversight process, but not normally subject to further or ongoing review, at the discretion of the entity responsible for the oversight process and subject to regulations and policies in the jurisdiction. Category 1B research includes the following activities:
Research that entails the in vitro formation of human stem cell-based embryo models that are not intended to represent the integrated development of the entire embryo including its extraembryonic membranes.
Scientists pursuing Category 1A and B research are recommended to consult with appropriate institutional review committees or the committee or body responsible for the specialized scientific and ethics review process (see Recommendation 2.1.3) to determine the categorization of new research proposals. Committees with the jurisdiction over the relevant research should oversee the provenance of cells, tissues and derived human pluripotent stem cell lines to ensure that procurement and derivation is deemed acceptable according to the principles outlined in this document (see section 2.3.5) and is in compliance with rigorous scientific, legal, and ethical standards.
2.2.2 Category 2
2.2.2 Category 2. Forms of research with embryos, certain chimeras, and stem cell-based embryo models that are permissible only after review and approval through a specialized scientific and ethics review process. A comprehensive review should be coordinated with other relevant oversight, such as that provided by human subjects review committees, in vitro fertilization (IVF) clinic oversight bodies, and animal research review processes (see 2.2.1), and the research should comply with local law and policy. All such research should have a compelling scientific rationale and necessitate the use of these materials rather than employ alternative models. The research should use the minimum number of embryos necessary to achieve the scientific objective. Forms of research requiring comprehensive review by a specialized review process encompass the following activities:
Generation of stem cell-based embryo models that represent the integrated development of the entire embryo including its extraembryonic membranes. These integrated stem cell-based embryo models should be maintained in culture for the minimum time necessary to achieve the scientific objective.
2.2.3 Category 3
2.2.3B Category 3B: Prohibited research activities. Research under this category should not be pursued because of broad international consensus that such experiments lack a compelling scientific rationale or are widely considered to be unethical. Such research includes:
Transfer of human stem cell-based embryo models to the uterus of either a human or animal host.
Public Representation of Science
Recommendation 4.1: The stem cell research community should promote accurate, current, balanced, and responsive public representations of stem cell research.
The high level of public and media interest in the field provides stem cell scientists with ample opportunities to communicate their findings through a variety of popular and social media channels. The research community is encouraged to responsibly engage interactively with the public through outreach and communications and by providing opportunities for public comment and feedback on scientific advances.
While such opportunities may allow scientists to gain recognition and understanding for their work among non-specialists, they also have the potential to fuel inaccurate public perceptions about the current state of scientific progress, potential for application, and associated risks and uncertainties (Kamenova and Caulfield, 2015). Scientists, clinicians, bioethicists, science communications professionals at academic and research institutions, and industry spokespersons should strive to ensure that benefits, risks, and uncertainties of stem cell science are not understated, misrepresented or overstated (see Recommendation 3.3.4.1). Additionally, due to public interest and concern in the ethics of human pluripotent stem cell research, and in order to ensure complete transparency of research and translational activities, the origin of stem cell materials should be clearly specified in all communications.
Care should be exercised throughout the science communication process, including in the promotion of research and translation activities, as well as in the presentation of scientific results, the use of social media, and in any communication with print and broadcast media. Particular caution should be exercised when preparing press releases and other types of promotional material. Researchers should make efforts to seek timely corrections of inaccurate or misleading public representations of research projects, achievements, or goals. Scientists should also be particularly careful about disclosing research findings that have not passed peer review, as premature reporting can undermine public confidence if findings are subsequently disproven. For example, if researchers post online preprints that have not been peer-reviewed, readers should be informed of the preliminary nature of such manuscripts.
Researchers must unintentionally avoid and correct inaccurate misconceptions in any communications regarding chimeras, genome editing, and other issues with a long history in the public imagination. While organoids, chimeras, embryo models, and other stem cell-based models are useful research tools offering possibilities for further scientific progress, limitations on the current state of scientific knowledge and regulatory constraints must be clearly explained in any communications with the public or media. Suggestions that any of the current in vitro models can recapitulate an intact embryo, human sentience or integrated brain function are unfounded overstatements that should be avoided and contradicted with more precise characterizations of current understanding. This is particularly relevant to brain organoids and human-animal chimeras, where any statements implying human cognitive abilities, human consciousness or self-awareness, as well as phrases or graphical representations suggesting human-like cognitive abilities risks misleading the public and sowing doubts about the legitimate nature of such research. Likewise, forward-looking statements on inherently uncertain developments, such as predictions on time required until clinical application, the likelihood of product approval, or speculation on the potential economic impact of currently unrealized technologies, must be accurate, circumspect, and restrained.
The stem cell community should work closely with communications professionals at their institution to create information resources that are easy to understand without oversimplifying, and that do not underplay risks and uncertainties or exaggerate potential benefits. Similarly, research-sponsoring institutions and communications professionals have a responsibility to ensure that any informational materials referring to research achievements adhere to these principles. Additionally, the scientists in charge of the research findings that are featured in informational materials should review and agree to the content prior to release. For potentially sensitive or high-profile cases, it is advisable to seek additional comments from independent experts to ensure objectivity and balance, place research in context of existing body of evidence, and help identify study limitations and alternative interpretations of key findings.
Glossary
Stem cell-based embryo models: Advances in cellular engineering make possible the assembly, differentiation, aggregation, or re-association of cell populations in a manner that models or recapitulates key stages of embryonic development. Such experimental systems can provide essential insights into embryo and tissue development but raise concerns when such structures achieve complexity to the point where they might realistically manifest the ability to undergo further integrated development if cultured for additional time in vitro. There are two types of stem cell-based embryo models.
Non-integrated stem cell-based embryo models: These stem cell-based embryo models will experimentally recapitulate some, but not all aspects of the peri-implantation embryo, for example differentiation of the embryonic sac or embryonic disc in the absence of extraembryonic cells. These stem cell-based embryo models do not have any reasonable expectations of specifying additional cell types that would result in formation of an integrated embryo model. Gastruloids are an example of a non-integrated stem cell-based embryo model.
Integrated stem cell-based embryo models: These stem cell-based embryo models contain the relevant embryonic and extra-embryonic structures and could potentially achieve the complexity where they might realistically manifest the ability to undergo further integrated development if cultured for additional time in vitro. Integrated stem cell-based embryo models could be generated from a single source of cells, for example expanded potential human pluripotent stem cells capable of coordinately differentiating into embryonic and extraembryonic structures. Alternatively, integrated stem cell-based embryo models could also be generated through the formation of cellular aggregates where extraembryonic/embryonic cells from one source are combined with embryonic/extraembryonic cells from different sources to achieve integrated human development. This might include using non-human primate cells as one of the sources. Previous restrictions on preimplantation human embryo culture (the “14-day/primitive streak rule”) were not written to apply to integrated stem cell-based embryo models. Thus, these guidelines specify the imperative for specialized review when such research is designed to model the integrated development of the entire embryo including its extraembryonic membranes. A guiding principle of review should be that the integrated stem cell-based embryo models should be used to address a scientific question deemed highly meritorious by a rigorous review process. Blastoids are an example of an integrated stem cell model.
-
This page assembles the sections of the Guidelines that specifically address research involving the transfer of human stem cells into animals. Other sections of the Guidelines may be relevant to such research (e.g., Section 2.1 Review Process and Section 2.3 Procurement and Informed Consent of Human Biological Materials).
2.2.1 Category 1
2.2.1A: Category 1A. Research determined to be exempt from a specialized scientific and ethics oversight process after being assessed by the appropriate existing mandates and committees for laboratory research. Category 1A research includes the following activities:
The transplantation of human stem cells, their derivatives, or other human cells into postnatal animal hosts (see Recommendation 2.2.1.1).
2.2.1B: Category 1B. Research that is reportable to the entity or body responsible for the specialized scientific and ethics oversight process, but not normally subject to further or ongoing review, at the discretion of the entity responsible for the oversight process and subject to regulations and policies in the jurisdiction. Category 1B research includes the following activities:
Chimeric embryo research in which human pluripotent stem cells are transferred into non-human, mammalian embryos and cultured in vitro for the minimum time necessary to achieve the scientific objective without gestation.
Scientists pursuing Category 1A and B research are recommended to consult with appropriate institutional review committees or the committee or body responsible for the specialized scientific and ethics review process (see Recommendation 2.1.3) to determine the categorization of new research proposals. Committees with the jurisdiction over the relevant research should oversee the provenance of cells, tissues and derived human pluripotent stem cell lines to ensure that procurement and derivation is deemed acceptable according to the principles outlined in this document (see section 2.3.5) and is in compliance with rigorous scientific, legal, and ethical standards.
Category 1B covers in vitro chimeric embryo research and in vitro gametogenesis for which there is no intent to generate a human embryo or fetus. Researchers are encouraged when possible to report on existing or planned in vitro experiments to the committee responsible for the specialized scientific and ethics oversight process to help identify cases that may warrant full review in the future.
Studies Transplanting Human Stem Cells or Their Direct Derivatives into the Central Nervous Systems of Animals
Recommendation 2.2.1.1: Research involving the transfer of human stem cells or their direct neural and/or glial derivatives into the central nervous systems of postnatal animal hosts requires review by institutional animal research oversight committees supplemented by reviewer expertise in stem cell or developmental biology. (ISSCR Guidelines, 2006; Academy of Medical Sciences, 2011). Such oversight should weigh the potential benefits of the research and should utilize available baseline non-human animal data grounded in rigorous scientific knowledge or reasonable inferences and involve a diligent application of animal welfare principles.
Institutions should determine whether research involving human cells that have the capacity to integrate into the nervous systems of laboratory animals requires supplementation of the pre-existing animal research review process with scientists and ethicists that have relevant topic-specific expertise.
To assist review and oversight of stem cell-based human-to-non-human animal research, the ISSCR has provided an advisory report that guides reviewers through a series of considerations not typically covered by institutional animal research committees, but that are relevant for review (Hyun et al., 2021). Past experiences with genetically altered laboratory animals have shown that reasonable caution might be warranted if changes carry the potential to produce new defects and deficits. Current best practices dictate that research involving modified animals must involve the following:
the establishment of baseline animal data;
ongoing data collection during research concerning any deviation from the norms of species-typical animals;
the use of small pilot studies to ascertain any changes in the welfare of modified animals; and
ongoing monitoring and reporting to animal research oversight committees authorized to decide the need for real-time changes in protocols and, if necessary, the withdrawal of animal subjects.
Research that may result in the presence of human gametes and their precursors in the gonads of laboratory animals is of not of significant ethical concern per se, as long as the animals are not allowed to breed (see Category 3 below).
Reviewers and investigators should follow the proposed ethical standards presented in the 2020 white paper and Appendix 1, while exercising appropriate judgment in individual situations. Research involving animals should also generally comply with the principles of the 3Rs (see: www.nc3rs.org.uk) and follow the ‘ARRIVE guidelines’ (Percie du Sert et. al 2020).
2.2.2 Category 2
2.2.2 Category 2. Forms of research with embryos, certain chimeras, and stem cell-based embryo models that are permissible only after review and approval through a specialized scientific and ethics review process. A comprehensive review should be coordinated with other relevant oversight, such as that provided by human subjects review committees, in vitro fertilization (IVF) clinic oversight bodies, and animal research review processes (see 2.2.1), and the research should comply with local law and policy. All such research should have a compelling scientific rationale and necessitate the use of these materials rather than employ alternative models. The research should use the minimum number of embryos necessary to achieve the scientific objective. Forms of research requiring comprehensive review by a specialized review process encompass the following activities:
Chimera research in which human pluripotent stem cells or their derivatives with broad potential are introduced into a) a non-human embryo or fetus in utero or b) a non-human embryo in vitro followed by transfer into a non-human uterus. Such experiments – if they are scientifically justified for the use of non-human primates above all other laboratory species – must exclude great and lesser ape species hosts (i.e., chimpanzees, gorillas, orangutans, bonobos, gibbons, and siamangs), as apes are prohibited from being used for invasive research in most parts of the world.
Human-Animal Chimeric Embryo Research
Recommendation 2.2.2.2: Chimeric embryo and in utero research described in ‘Category 2, i’ (see above) should proceed for the minimum time necessary to achieve the scientific aim. This research must proceed incrementally, stopping at well-defined timepoints to assess the degree and scope of chimerism during development before proceeding to full gestation, if full gestation is among the well-justified goals of the research. To avoid unpredictable and widespread chimerism, researchers should endeavor to use targeted chimerism strategies to limit chimerism to a particular organ system or region of the gestating chimeric animal.
Techniques such as blastocyst complementation, whereby a specific cell type or organ is effectively deleted as the host embryo develops, can lead to a specific cell type or organ being replaced entirely by derivatives from the donor-derived pluripotent stem cells. By itself this targeted chimerism may not prevent contributions elsewhere in the chimera, thus the need for an incremental approach. Nonetheless, if the host cells have an advantage over the donor cells, such as even a slightly faster rate of cell replication, then the donor cells will be disadvantaged and effectively selected against, leading to a little or no contribution outside the organ of choice.
As a general principle, non-human primate species should only be used when all other species, more distant in evolution from humans, are inadequate for the scientifically well justified research question being pursued. Appropriate research aims include understanding human development, understanding species barriers to chimerism, and treating disease. Any research involving non-human primates must utilize common laboratory species that are widely used in biomedical research (which excludes apes). Trained veterinary staff specializing in the care of non-human primates must be closely involved in the review and oversight of studies involving the transfer of human stem cells and their derivatives into nonhuman primate hosts.
2.2.3 Category 3
2.2.3B Category 3B: Prohibited research activities. Research under this category should not be pursued because of broad international consensus that such experiments lack a compelling scientific rationale or are widely considered to be unethical. Such research includes:
Research in which animal chimeras incorporating human cells with the potential to form human gametes are bred to each other.
Transfer of chimeric embryos mixing animal and human cells (whether predominantly animal or human) to the uterus of a human or great or lesser ape (i.e., chimpanzees, gorillas, orangutans, bonobos, gibbons, and siamangs).
Public Representation of Science
Recommendation 4.1: The stem cell research community should promote accurate, current, balanced, and responsive public representations of stem cell research.
The high level of public and media interest in the field provides stem cell scientists with ample opportunities to communicate their findings through a variety of popular and social media channels. The research community is encouraged to responsibly engage interactively with the public through outreach and communications and by providing opportunities for public comment and feedback on scientific advances.
While such opportunities may allow scientists to gain recognition and understanding for their work among non-specialists, they also have the potential to fuel inaccurate public perceptions about the current state of scientific progress, potential for application, and associated risks and uncertainties (Kamenova and Caulfield, 2015). Scientists, clinicians, bioethicists, science communications professionals at academic and research institutions, and industry spokespersons should strive to ensure that benefits, risks, and uncertainties of stem cell science are not understated, misrepresented or overstated (see Recommendation 3.3.4.1). Additionally, due to public interest and concern in the ethics of human pluripotent stem cell research, and in order to ensure complete transparency of research and translational activities, the origin of stem cell materials should be clearly specified in all communications.
Care should be exercised throughout the science communication process, including in the promotion of research and translation activities, as well as in the presentation of scientific results, the use of social media, and in any communication with print and broadcast media. Particular caution should be exercised when preparing press releases and other types of promotional material. Researchers should make efforts to seek timely corrections of inaccurate or misleading public representations of research projects, achievements, or goals. Scientists should also be particularly careful about disclosing research findings that have not passed peer review, as premature reporting can undermine public confidence if findings are subsequently disproven. For example, if researchers post online preprints that have not been peer-reviewed, readers should be informed of the preliminary nature of such manuscripts.
Researchers must unintentionally avoid and correct inaccurate misconceptions in any communications regarding chimeras, genome editing, and other issues with a long history in the public imagination. While organoids, chimeras, embryo models, and other stem cell-based models are useful research tools offering possibilities for further scientific progress, limitations on the current state of scientific knowledge and regulatory constraints must be clearly explained in any communications with the public or media. Suggestions that any of the current in vitro models can recapitulate an intact embryo, human sentience or integrated brain function are unfounded overstatements that should be avoided and contradicted with more precise characterizations of current understanding. This is particularly relevant to brain organoids and human-animal chimeras, where any statements implying human cognitive abilities, human consciousness or self-awareness, as well as phrases or graphical representations suggesting human-like cognitive abilities risks misleading the public and sowing doubts about the legitimate nature of such research. Likewise, forward-looking statements on inherently uncertain developments, such as predictions on time required until clinical application, the likelihood of product approval, or speculation on the potential economic impact of currently unrealized technologies, must be accurate, circumspect, and restrained.
The stem cell community should work closely with communications professionals at their institution to create information resources that are easy to understand without oversimplifying, and that do not underplay risks and uncertainties or exaggerate potential benefits. Similarly, research-sponsoring institutions and communications professionals have a responsibility to ensure that any informational materials referring to research achievements adhere to these principles. Additionally, the scientists in charge of the research findings that are featured in informational materials should review and agree to the content prior to release. For potentially sensitive or high-profile cases, it is advisable to seek additional comments from independent experts to ensure objectivity and balance, place research in context of existing body of evidence, and help identify study limitations and alternative interpretations of key findings.
-
This page assembles the sections of the Guidelines that specifically address research involving heritable genome editing. Other sections of the Guidelines may be relevant to such research (e.g., Section 2.1 Review Process, Section 2.3 Procurement and Informed Consent of Human Biological Materials, and Chapter 3. Clinical Translation of Stem Cell-based Interventions).
2.2.2 Category 2
2.2.2 Category 2. Forms of research with embryos, certain chimeras, and stem cell-based embryo models that are permissible only after review and approval through a specialized scientific and ethics review process. A comprehensive review should be coordinated with other relevant oversight, such as that provided by human subjects review committees, in vitro fertilization (IVF) clinic oversight bodies, and animal research review processes (see 2.2.1), and the research should comply with local law and policy. All such research should have a compelling scientific rationale and necessitate the use of these materials rather than employ alternative models. The research should use the minimum number of embryos necessary to achieve the scientific objective. Forms of research requiring comprehensive review by a specialized review process encompass the following activities:
Research involving the genetic alteration of human embryos or gametes used to make embryos in vitro.
2.2.3 Category 3
2.2.3A Category 3A. Research activities currently not permitted. Research under this category should not be pursued at this time because the approaches are currently unsafe or raise unresolved ethical issues. There may be valid reasons for undertaking the research in the future, but this should not proceed until the safety and ethical issues are resolved. Such research includes:
Research in which human embryos that have undergone modification to their nuclear genome are transferred into or gestated in a human uterus. Genome-modified human embryos include human embryos with engineered alterations to their nuclear DNA and embryos generated from a human gamete that has had its nuclear DNA modified, when such modifications could be inherited through the germline. While there are valid reasons for pursuing this line of research, which may include situations where correcting a deleterious gene variant is the only way that prospective parents may have a genetically-related child (National Academy of Medicine, National Academy of Sciences, and the Royal Society, 2020), conduct of such research will be dependent on appropriate policies, regulations, and oversight.
2.2.3B Category 3B: Prohibited research activities. Research under this category should not be pursued because of broad international consensus that such experiments lack a compelling scientific rationale or are widely considered to be unethical. Such research includes:
Research in which human embryos produced by reprogramming of nuclei are implanted into a human or animal uterus (often referred to as human reproductive cloning).
Emerging Categories of Embryo Research That Merit Close Review: Heritable Genome Editing
Recommendation 2.2.3.1: Until there is further scientific clarity regarding how to achieve desired genetic alterations, additional evidence for safety, and wider discussion and consensus on ethics (i.e., whether it should be done and, if so, under which circumstances), any attempt to edit the mitochondrial genome or modify the nuclear genome of human embryos for the purpose of human reproduction is premature and should not be permitted at this time (see Section 2.2.3A, Category 3A, a).
Preclinical research that entails modifying the nuclear genomes of gametes, zygotes, and human embryos may be permissible under a rigorous specialized oversight process (Category 2). Such research promises to enhance fundamental knowledge and is essential to inform deliberations about the potential safety and use of nuclear or mitochondrial DNA genome editing in strategies aimed at preventing the transmission of serious genetic disorders.
Scientists currently lack an adequate understanding of the fidelity and precision of techniques for genome editing of human embryos, as well as a full appreciation of the safety, ethics, and potential long-term risks and benefits to individuals born following such a process. This is described in more detail in the recent report, Heritable Human Genome Editing, from the International Commission on the Clinical Use of Human Genome Editing (National Academy of Medicine, National Academy of Sciences, and the Royal Society, 2020), which suggests a responsible translational pathway in certain circumstances, albeit one that cannot currently be met. Of note, the focus of this report was on developing a responsible translational pathway; it did not include a broad look at the societal and ethical issues, as these were outside its task. Such issues have been considered elsewhere (e.g. by the Nuffield Council on Bioethics). The forthcoming report from the WHO Advisory Committee on Developing Global Standards for Governance and Oversight of Human Genome Editing will bear in mind societal views and ethical principles, but this will focus on mechanisms of governance.
Basic and preclinical research is needed to minimize the potential harms resulting from intended and unintended edits, which could be passed to future generations, as well as direct or indirect effects of the editing process that could affect embryo viability or developmental potential.
3.4.8 Clinical Research that Involves Heritable Changes to the Human Genome
Heritable Genome Editing
Recommendation 3.4.8.3.1: Substantial preclinical research is needed to minimize the potential harm associated with clinical applications involving heritable genome editing; therefore, any attempt to modify the nuclear genome of human embryos for the purpose of reproduction is premature and should not be permitted at this time (see Section 2.1.3.3, Category 3A, a).
Any decision to proceed with heritable genome editing, where modified human embryos are transferred into a uterus or otherwise allowed to develop in utero, must be preceded with adequate preclinical research to minimize the potential harms from intended and unintended edits (see Recommendation 2.1.4). The first-in-human clinical uses should only be considered for the most favorable balance of potential harms and benefits and this will be most clearly defined for diseases and patients for which there are no viable alternatives. This may include prospective parents for whom there are no or very limited available alternatives for preventing transmission of diseases and conditions for which mortality is high and morbidity is severe. Other options for having a healthy child, including adoption, gamete or embryo donation, and preimplantation genetic testing, should be considered with appropriate counselling prior to any decision to proceed.
Recommendation 3.4.8.3.2: If the technical and safety challenges associated with human heritable editing are resolved (see Recommendations 2.1.4 and 3.4.8.3.1), any applications for the initial clinical use of human heritable genome editing should be evaluated on a case-by-case basis. This evaluation needs to consider not just the scientific methods, but also the societal and ethical issues associated with the proposed use.
The decision to proceed with first-in-human clinical uses needs to be taken openly with robust consideration of informed public opinion generated through meaningful public engagement. In addition, and critically, any experimental use of heritable human genome editing should only proceed in jurisdictions with appropriate and robust regulations and oversight.
A key consideration of potential uses of heritable genome editing is whether the prospective parents have feasible options for conceiving a genetically related child who does not inherit a serious genetic disease, such as preimplantation genetic testing and selection of embryos. The initial uses should be confined to prospective parents who lack reasonable alternatives.
It is important that the biological consequences of the intended genome edit are well understood, both for the immediate offspring and for future generations who might inherit it, in order to minimize the potential for an intended edit to have unintended deleterious consequences (on its own, via genetic interactions with other loci, or via environmental interactions). At present, the best way to achieve this goal is to use editing to change a known pathogenic genetic variant to one that is present in unaffected family members, common in the relevant population, or known not to be disease-causing.
Recommendation 3.4.8.3.3: A comprehensive regulatory and ethical framework for overseeing heritable genome editing must be established before any first-in-human clinical applications are considered. This framework should build on the existing regulatory frameworks for new biotechnologies, the practice of medicine, and the principles outlined in these guidelines (see Section 3.3 and 3.4).
The regulatory framework for heritable genome editing must ensure that there is robust multi-generational follow-up to identify adverse reactions that may occur due to inherited genome alterations. However, this needs to be done in such a way as to protect the confidentiality of the prospective parents and any children born. The framework must ensure that there is a robust informed consent process that builds on the informed consent process discussed in these guidelines (see recommendations 3.4.2.5 and 3.4.4.1) and includes a discussion of potential alternative treatments (if any) and the multigenerational risks and benefits of pregnancies involving the implantation of germline genome edited embryos, including those derived from genetically modified gametes.
Recommendation 3.4.8.3.4: Regulators, research funders, and academic and medical societies should seek to prevent the premature or unethical clinical uses of heritable genome editing unless and until the safety, ethical, and societal issues associated with the clinical use of heritable genome editing are resolved.
It is incumbent upon the entire biomedical research community to monitor for potential unethical and premature clinical uses of human heritable genome editing technologies. Researchers are strongly encouraged to report potential unethical uses to regulators, funders, licensing bodies, and academic societies to evaluate potential unethical uses of this technology.
-
This page assembles the sections of the Guidelines that specifically address research involving mitochondrial replacement techniques. Other sections of the Guidelines may be relevant to such research (e.g., Section 2.1 Review Process, Section 2.3 Procurement and Informed Consent of Human Biological Materials, and Chapter 3. Clinical Translation of Stem Cell-based Interventions).
2.2.2 Category 2
2.2.2 Category 2. Forms of research with embryos, certain chimeras, and stem cell-based embryo models that are permissible only after review and approval through a specialized scientific and ethics review process. A comprehensive review should be coordinated with other relevant oversight, such as that provided by human subjects review committees, in vitro fertilization (IVF) clinic oversight bodies, and animal research review processes (see 2.2.1), and the research should comply with local law and policy. All such research should have a compelling scientific rationale and necessitate the use of these materials rather than employ alternative models. The research should use the minimum number of embryos necessary to achieve the scientific objective. Forms of research requiring comprehensive review by a specialized review process encompass the following activities:
Transferring human embryos to a human uterus following mitochondrial replacement.
Mitochondrial Replacement Techniques
Recommendation 2.2.2.3: Further research should be undertaken to refine and assess the safety and efficacy of Mitochondrial Replacement Techniques (MRT), including minimizing a) the risk of mitochondrial carryover and b) disruptions to the interaction between mitochondrial and nuclear genomes. In addition, further research on polar body transfer techniques and the use of mitophagy or genome editing is needed to reduce or eliminate pathogenic mitochondrial DNA. Such research should be subject to review by a specialized oversight process as Category 2 Research (Section 2.1.5.2).
MRT is being explored to prevent the transmission of serious mitochondrial DNA-based diseases in at-risk pregnancies (see Section 3.4.8). MRT most commonly involves transferring the nuclear DNA from the prospective mother’s oocyte or fertilized oocyte (pronuclear stage) to that of a mitochondrial donor from which the nuclear DNA has been removed [maternal spindle transfer (MST) or pronuclear transfer (PNT), respectively]. The mitochondrial donor is selected to be free of known pathogenic mutations. Input from clinicians and scientists with relevant expertise in mitochondrial and embryo biology should augment the review process for evaluating clinical protocols that entail uterine transfer of human embryos for the purpose of human reproduction.
2.2.3 Category 3
2.2.3A Category 3A. Research activities currently not permitted. Research under this category should not be pursued at this time because the approaches are currently unsafe or raise unresolved ethical issues. There may be valid reasons for undertaking the research in the future, but this should not proceed until the safety and ethical issues are resolved. Such research includes:
Research in which human embryos that have undergone editing to their mitochondrial genome are transferred into or gestated in a human uterus, as current knowledge of such interventions is inadequate to ensure safety.
3.4.8 Clinical Research that Involves Heritable Changes to the Human Genome
Mitochondrial Replacement Techniques
Recommendation 3.4.8.1: Mitochondrial Replacement Techniques (MRT) should be offered only in the context of clinical investigation that is subject to strict regulatory oversight, limited to patients at high risk of transmitting serious mitochondrial DNA-based diseases to their offspring, when no other treatments are acceptable, and where long-term follow-up is feasible. International data sharing arising from initial uses is essential to help inform the field and ensure its appropriate use.
Initial applications of MRT should continue to be restricted to cases in which the probability of transmission of pathogenic mitochondrial DNA is very high, where preimplantation genetic testing is unlikely to identify embryos suitable for transfer, and where procedures are conducted in the context of clinical investigation that can contribute to generalizable knowledge about this currently unproven and experimental technique. Preclinical research in human embryo-derived stem cells following MRT indicated the possibility of levels of maternal mitochondrial DNA increasing with extended passaging, but the clinical relevance of these data is unclear. Concerns have also been expressed about the possibility of mito-nuclear interactions being disrupted by MRT, though these remain theoretical. Research on embryonic stem cells derived from embryos after MRT or on the embryos themselves maintained in vitro would help explore these issues. These experiments could only proceed in jurisdictions where the creation of embryos for research is allowed, and only where they have been permitted following review by a specialized oversight process (see Section 2.1).
Recommendation 3.4.8.2: There are inadequate clinical and preclinical data to justify the use of MRT to treat unexplained infertility associated with poor oocyte/embryo quality in women; therefore, it is recommended that this not be an intervention at this time.
MRT has been used in the clinic as a speculative treatment for infertility (Zhang et al 2016). Given the risks entailed and the absence of clear mechanisms and compelling rationale for the use of MRT for unexplained infertility, additional preclinical and clinical experience are required to establish safety and efficacy. In one reported pilot trial of MRT, embryonic development and pregnancy rates in patients of advanced maternal age did not increase (n= 30), and it was advised that such patients should not undergo MRT (Mazur, 2019). Data from another small (non-randomized) trial of MRT (n= 25), in women under 40 with previous multiple failures of IVF (Costa-Borges et al, 2020), suggest that further controlled trials and follow-up are required. Research conducted in vitro to understand the mechanism by which application of the techniques may help unexplained infertility (which might not involve mitochondria) should be conducted, notably because this may lead to alternatives that both circumvent the use of technically challenging methods and avoid any risks associated with heteroplasmy or disrupted mito-nuclear interactions.
-
This page assembles the sections of the Guidelines that specifically address research involving organoids. Other sections of the Guidelines may be relevant to such research (e.g., Section 2.3 Procurement and Informed Consent of Human Biological Materials).
2.2.1 Category 1
2.2.1A: Category 1A. Research determined to be exempt from a specialized scientific and ethics oversight process after being assessed by the appropriate existing mandates and committees for laboratory research. Category 1A research includes the following activities:
Research on stem cell culture systems that model specific stages of development or specific anatomic structures rather than the continuous development of an intact embryo or fetus. These would include but are not restricted to models of amnion formation, neural tube development, development of primordial germ cells, placental structures, 2D or 3D models of gastrulation or post-gastrulation events, and in vitro stem cell-derived organoids in culture that recapitulate most aspects of organ function, but not those that fall under subsequent categories.
Organoid Research
At this time, there is no biological evidence to suggest any issues of concern, such as consciousness or pain perception with organoids corresponding to CNS tissues, that would warrant review through the specialized oversight process. However, researchers should be aware of any ethical issues that may arise in the future as organoid models become more complex through long-term maturation or through the assembly of multiple organoids (Hyun et al. 2020).
Public Representation of Science
Recommendation 4.1: The stem cell research community should promote accurate, current, balanced, and responsive public representations of stem cell research.
The high level of public and media interest in the field provides stem cell scientists with ample opportunities to communicate their findings through a variety of popular and social media channels. The research community is encouraged to responsibly engage interactively with the public through outreach and communications and by providing opportunities for public comment and feedback on scientific advances.
While such opportunities may allow scientists to gain recognition and understanding for their work among non-specialists, they also have the potential to fuel inaccurate public perceptions about the current state of scientific progress, potential for application, and associated risks and uncertainties (Kamenova and Caulfield, 2015). Scientists, clinicians, bioethicists, science communications professionals at academic and research institutions, and industry spokespersons should strive to ensure that benefits, risks, and uncertainties of stem cell science are not understated, misrepresented or overstated (see Recommendation 3.3.4.1). Additionally, due to public interest and concern in the ethics of human pluripotent stem cell research, and in order to ensure complete transparency of research and translational activities, the origin of stem cell materials should be clearly specified in all communications.
Care should be exercised throughout the science communication process, including in the promotion of research and translation activities, as well as in the presentation of scientific results, the use of social media, and in any communication with print and broadcast media. Particular caution should be exercised when preparing press releases and other types of promotional material. Researchers should make efforts to seek timely corrections of inaccurate or misleading public representations of research projects, achievements, or goals. Scientists should also be particularly careful about disclosing research findings that have not passed peer review, as premature reporting can undermine public confidence if findings are subsequently disproven. For example, if researchers post online preprints that have not been peer-reviewed, readers should be informed of the preliminary nature of such manuscripts.
Researchers must unintentionally avoid and correct inaccurate misconceptions in any communications regarding chimeras, genome editing, and other issues with a long history in the public imagination. While organoids, chimeras, embryo models, and other stem cell-based models are useful research tools offering possibilities for further scientific progress, limitations on the current state of scientific knowledge and regulatory constraints must be clearly explained in any communications with the public or media. Suggestions that any of the current in vitro models can recapitulate an intact embryo, human sentience or integrated brain function are unfounded overstatements that should be avoided and contradicted with more precise characterizations of current understanding. This is particularly relevant to brain organoids and human-animal chimeras, where any statements implying human cognitive abilities, human consciousness or self-awareness, as well as phrases or graphical representations suggesting human-like cognitive abilities risks misleading the public and sowing doubts about the legitimate nature of such research. Likewise, forward-looking statements on inherently uncertain developments, such as predictions on time required until clinical application, the likelihood of product approval, or speculation on the potential economic impact of currently unrealized technologies, must be accurate, circumspect, and restrained.
The stem cell community should work closely with communications professionals at their institution to create information resources that are easy to understand without oversimplifying, and that do not underplay risks and uncertainties or exaggerate potential benefits. Similarly, research-sponsoring institutions and communications professionals have a responsibility to ensure that any informational materials referring to research achievements adhere to these principles. Additionally, the scientists in charge of the research findings that are featured in informational materials should review and agree to the content prior to release. For potentially sensitive or high-profile cases, it is advisable to seek additional comments from independent experts to ensure objectivity and balance, place research in context of existing body of evidence, and help identify study limitations and alternative interpretations of key findings.
-
Oversight
Recommendation 2.1.1: All research that (a) involves preimplantation stages of human development, in vitro human embryo culture, derivation of new embryo-derived cells or lines, integrated stem cell-based embryo models, or (b) entails the production of human gametes in vitro when such gametes are tested by fertilization or used for the creation of embryos, shall be subject to review, approval, and ongoing monitoring, as appropriate, through a specialized oversight process capable of evaluating the unique aspects of the science and the associated ethical issues (see below).
The specialized scientific and ethics oversight process encompasses the review of human embryo and related stem cell research. The process can be performed at the institutional, local, regional, national, or international level or by some coordinated combination of those elements and need not be served by a single, specific committee, so long as the oversight process as a whole occurs effectively, impartially, and rigorously. Provided appropriate expertise is available to ensure that the scientific, ethical, and legal aspects of the research can be rigorously evaluated, the specialized oversight can occur through preexisting institutional review processes that assess the participation of human subjects in research, the procurement of human tissues in and for research, or biosafety and ethical issues associated with research. For example, existing review bodies such as the Embryonic Stem Cell Research Oversight (ESCRO; Institute of Medicine and National Research Council, 2005), Stem Cell Research Oversight (SCRO; ISSCR Guidelines, 2006), or Embryo Research Oversight (EMRO; ISSCR Guidelines 2016) committees in the U.S., or the UK HFEA and regional ethics committees (RECs), are well positioned to perform review and oversight of embryo and related research. A single rather than redundant review is preferable as long as this is thorough and capable of addressing any uniquely sensitive elements of human embryo research and hESC research.
Recommendation 2.1.2: The specialized scientific and ethics oversight process must include an assessment of the scientific rationale and merit of research proposals, the relevant expertise of the researchers, and the ethical permissibility and justification for the research as discussed below.
Scientific rationale and merit of the proposal: Research involving human embryonic cells or human embryos and gametes requires that scientific goals and methods be scrutinized to ensure scientific rigor. Adequate and appropriate scientific justification for performing the research using the specified materials is required.
Relevant expertise of researchers: Appropriate expertise and training of the researchers to perform the stated experiments must be ascertained in order to ensure the appropriate use of research materials. For the derivation of new human embryo-derived cell lines, the formation of human embryo models from stem cells, or experiments that involve the use of human embryos, relevant expertise would include prior experience with embryo culture and stem cell derivation in animal systems and competence in the culture and maintenance of human embryonic stem cells. Researchers performing derivations of embryo-derived cell lines should have a detailed, documented plan for characterization, storage, banking, and distribution of new lines.
Ethical permissibility and justification: Research goals must be assessed within an ethical framework to ensure that research proceeds in a transparent and responsible manner. The project proposal should include a discussion of alternative methods and provide a rationale for performing the experiments in a human rather than animal model system, for the proposed methodology, and if the studies involve preimplantation human embryos, a justification for the anticipated numbers to be used.
Recommendation 2.1.3: The committee or body conducting the specialized scientific and ethics oversight process is responsible for (a) advising researchers on the categorization of research (see Recommendation 2.2), (b) determining whether a research proposal constitutes permissible or non-permissible research, (c) monitoring and periodically reviewing ongoing research, and (d) overseeing the provenance of the human pluripotent stem cell lines used in Category 2 Research (see section 2.2.2).
The responsible committee or entity should interpret these guidelines, define research practices, and monitor compliance. Researchers are encouraged to consult the committee or entity for advice on how to determine whether research is exempt as Category 1A (see 2.2.1)
Composition of Research Review and Oversight Bodies
Recommendation 2.1.4: The specialized scientific and ethics oversight process should be conducted by qualified scientists, ethicists, legal and regulatory experts, and community members who are not directly engaged in the research under consideration. The oversight process must include participants with the following perspectives:
Scientists and/or physicians with relevant expertise, including representation from scientists that are not directly engaged in the research under consideration. Relevant expertise includes areas of stem cell biology, assisted reproduction, developmental biology, and clinical medicine.
Ethicists with the ability to interpret the ethical justifications for, and implications of, the research under consideration.
Those familiar with relevant local policies and statutes governing the research.
Community members, unaffiliated with the institution where the research is conducted through employment, who are impartial and reasonably familiar with the views and needs of patients and patient communities who could be benefited by stem cell research, and community standards.
Additional members with relevant expertise not already represented on the oversight body should be included as required, for example to cover research involving human genetics, physiology, molecular biology, etc.
The policies and regulations of each country or jurisdiction will determine whether the specialized scientific and ethics oversight process will be performed by internal or external bodies at the institutional or national level. Participants in the specialized scientific and ethics oversight process should be selected based on their relevant area-specific expertise (e.g., scientific, clinical, ethics, research policy). Those engaged in the oversight process must be cognizant of potential financial and non-financial conflicts of interest that might compromise the integrity of the review. Such conflicts must be disclosed, evaluated, and minimized, or eliminated as much as possible.
-
2.3 Procurement and Informed Consent of Human Biological Materials
The procurement of human gametes, embryos, fetal tissues, and somatic cells is integral to the conduct of human embryo and stem cell research. Human biological materials must be procured in accordance with generally accepted principles of research ethics and laws and policies in their respective jurisdictions.
2.3.1 Review Process for the Procurement of Human Cells and Tissues
Recommendation 2.3.1. The review process for the procurement of human cells and tissues should be predicated on the source of the material and its intended use as described in the three tiers below.
Tier 1. Banked and historical cell lines. The procurement of cell lines from repositories or banks is permissible if the materials have been deposited and distributed consistent with the original consent for use of the donated human cells and tissues and these guidelines (see Section 2.4, Derivation, Banking and Distribution of Human Stem Cell Lines) and the standards at the time (Sugarman et al. 2008). Toward this end, the repositories or banks should require certification from depositors confirming the ethical provenance of such cells, including consent and ethics approvals. The use of historical cell lines from pathological specimens, such as HeLa, are permissible for use in stem cell research that is otherwise compliant with these guidelines. Likewise, stem cell lines procured from vendors are permissible for stem cell research, provided the vendor generated and distributed the stem cell lines in a manner consistent with the original donor consent and contemporaneous ethical and regulatory standards. The procurement of Tier 1 cell lines should not be used for reproductive purposes.
Tier 2. Fresh human somatic cells and tissues. The procurement of fresh human somatic cells and tissues for stem cell research purposes should be reviewed by existing research review committees bolstered by stem cell specific expertise in accordance with generally accepted principles of research ethics and laws and regulations in the respective jurisdiction and these guidelines (see Sections 2.3.2 and 2.3.3).
Tier 3. Gametes and embryos. The procurement of human gametes and embryos that are destined for use in human embryo research and stem cell research must be reviewed through the specialized oversight process and existing research review committees in accordance with generally accepted principles of research ethics and laws and regulations in the respective jurisdiction and these guidelines (see Sections 2.3.2 and 2.3.3).
Review by a specialized oversight process (Tier 3) or existing research review committee bolstered by stem cell-specific expertise must ensure that vulnerable populations are not exploited due to their dependent status or any compromised ability to offer voluntary consent and that there are no undue inducements or other undue influences for the provision of human cells and tissues.
2.3.2 Informed Consent for the Donation of Human Cells and Tissues
Recommendation 2.3.2.1: Embryos, fetal tissue, and other cells and tissues should be used in research only if voluntary informed consent was obtained from the donors before the research commences. The informed consent process should be robust and document the prospect of therapeutic and commercial applications as well as the potential research uses, such as the creation of hESCs, iPSCs, other immortalized cell lines, embryos, and gametes. In the case of fetal tissue, consent from the woman donating the tissue is sufficient. In the case of embryos made with donor gametes, this consent should be obtained from the gamete donors and the party(ies) with authorization to donate the embryo.
Most patients and research subjects may donate cells or tissues with broad consent to a range of future uses; however, the broad consent does not apply to use of donated cells and tissues for reproductive purposes. The consent may be obtained at the time of tissue collection or with a re-contact for additional consent to use donated cells and tissues for reproductive purposes.
In the case that human cells and tissues are procured from a minor or adult that lacks the decision-making capacity to provide informed consent, consent must be provided by a parent, legal guardian, or other legally authorized person. Whenever feasible, the assent of the minor or decisionally incapacitated adult is also strongly encouraged.
Empirical research has shown that informed consent is most effective as a dynamic, interactive, and evolving process as opposed to a static, one-time disclosure event (Flory and Emanuel, 2004). The informed consent document alone can never take the place of a meaningful dialogue between the person obtaining consent and the donors of human cells and tissues. The informed consent process can be enhanced in the following ways:
Whenever possible, the person conducting the informed consent dialogue should have no vested interest in the research protocol. If members of the research team participate in the informed consent process, their role and any other potential conflicts of interest must be disclosed, and care taken to ensure that information is provided in a transparent, accurate, unbiased manner.
The person conducting the informed consent process should provide ample opportunities for cell and tissue donors to ask questions and discuss their involvement in the research protocol.
Counseling services should be made available upon request to any potential providers of human cells and tissues prior to procurement.
Consent processes and documents should be revised in light of new research on informed consent for all types of human biological materials procurement and where relevant, ongoing studies of the long-term risks associated with oocyte retrieval.
Separating Research Consent from Treatment
Recommendation 2.3.2.2: Informed consent for research use must be distinct from informed consent for clinical treatment.
Decisions related to the provision of gametes or the creation of embryos for fertility treatment should be a voluntary choice that is free from undue influence by researchers who propose to use these cells in research. During the course of clinical treatment, researchers may not request that members of the fertility treatment team generate more embryos or harvest more oocytes than necessary for the patient’s optimal fertility treatment. Wherever possible, the treating infertility clinician should not be the investigator who is proposing to perform research on the procured materials.
Consistent with fetal tissue research guidelines issued by the Network of European CNS Transplantation and Restoration (NECTAR) and U.S. regulations, a woman’s decision to terminate a pregnancy must not be influenced by the possible research use of her fetus’ tissues (Boer, 1994; OHRP, 1993). Informed consent for fetal tissue procurement and research should be obtained from the woman only after her decision to legally terminate her pregnancy but before the abortion procedure, or after a spontaneous abortion. Medical procedures must not put the woman at any increased risk solely to facilitate the research use of donated fetal tissues. Clinicians obtaining informed consent and clinics at which informed consent is sought may not profit from the procurement of fetal tissues for research.
Review of Cell and Tissue Collection for Embryo and Stem Cell Research
Recommendation 2.3.2.3: Review of procurement protocols must ensure that cell and tissue donors are adequately informed about the specific aspects of their voluntary research participation.
Researchers should exercise care in seeking and obtaining informed consent from prospective donors. The informed consent process should take into account language barriers, the educational level and reading comprehension level of the research subjects, and any other impediments to good communication. Empirical research has shown that comprehension during the informed consent process improves with the use of interactive methods interaction (Flory and Emanuel, 2004). To facilitate the adoption of adequate and uniform standards of informed consent for the procurement of cells and tissues for research, the ISSCR provides template documents that can be downloaded and customized to specific protocols (Appendix 2). These sample documents will need to be customized for use in specific research studies and conform to local laws and policies.
If pluripotent stem cells are to be derived from procured cells or tissues, the informed consent document and discussion should cover information that addresses key aspects of human stem cell research, including but not limited to the fact that an immortal stem cell line could be established that is a partial or full genetic match to the cell or tissue donor and that the stem cell line could be shared with other researchers outside the institution and jurisdiction for other research purposes that may not be fully anticipated at this time. For a list of informed consent discussion points, see Appendix 3.
Incidental Findings
Recommendation 2.3.2.4: Researchers should develop a policy that states whether and how incidental findings will be provided to cell and tissue donors. This policy must be explained during the informed consent process. Cell and tissue donors should be able to choose whether they wish to receive incidental findings, if any. Reporting findings with relevance to public health may be required by law in certain jurisdictions.
During the course of research with human stem cell lines, particularly lines derived from somatic cells, investigators may discover information that may be of importance to cell and tissue donors, such as BRCA1/2 mutations. Because the net harms and benefits of disclosing incidental findings to cell and tissue donors are presently unclear, a single approach to managing incidental findings may not be appropriate across all studies and jurisdictions. When studies include a plan to disclose incidental findings to research subjects, researchers must offer a practical and adequately resourced feedback mechanism that involves donors’ physicians and, where possible, the verification of any discovered incidental findings.
For a given sample, secondary researchers should adhere to the incidental findings policies that were developed by the primary researchers (or others collecting cells and tissues) and disclosed to donors during the informed consent process.
If re-contact is required, instructions on how to report incidental findings (report to provider, researcher, institution, physician, etc.) should be specified in the material transfer agreement. Re-contact is a matter for primary research sites to manage. However, secondary researchers should be aware of the incidental findings policies of either of these responsible parties.
Successful implementation of a policy on incidental findings depends crucially on the traceability of cell line distribution. Therefore, all providers and recipients should ensure that cell lines are used under strict compliance with material transfer agreements and the regulations in the informed consent process.
Consent for De-identified Cells and Tissues
Recommendation 2.3.2.5: Researchers are encouraged to discuss the potential for genomic sequencing to connect de-identified cells and tissues to donors and their relatives during the informed consent process for the donation.
Cells and tissues donated for research are often de-identified to protect the privacy of donors. Due to advances in genomic sequencing, it may be possible for researchers to connect de-identified cell and tissue samples with donors or their relatives. Researchers are encouraged to require confidentiality when sharing genomic data that has the potential to connect donors and family members with de-identified cells and tissues.
2.3.3 Payments to Individuals Donating Cells and Tissue for Research
Recommendation 2.3.3.1: Research oversight committees must authorize all proposals to reimburse for out-of-pocket expenses to donors of embryos, sperm, or somatic cells.
Individuals who choose to provide previously stored cells and tissues for research should not be reimbursed for the costs of storage prior to the decision to participate in research. For the provision of fresh somatic cells or sperm for research, reimbursement for out-of-pocket expenses incurred by donors may be determined during the review process. For the provision of embryos or fetal tissue for research, no payment or valuable consideration of any kind beyond reimbursement of out-of-pocket expenses may be offered to donors for their procurement.
Recommendation 2.3.3.2: For the provision of oocytes for research, when oocytes are collected outside the course of clinical treatment, compensation for non-financial burdens should not constitute an undue inducement.
Because women carry particular burdens during the procurement of their gametes, their efforts should be acknowledged fairly and appropriately. At the same time, precaution is needed to avoid the potential for exploitation.
In jurisdictions where the provision of oocytes for research is legally permissible, the human subjects review committee and those responsible for conducting specialized research oversight must assess the safety and the voluntary and informed choice of women to provide oocytes for research according to the following standards:
There should be monitoring of recruitment practices of oocyte donors to ensure that the decision of women to donate their oocytes is free of undue inducement and exploitation.
In jurisdictions where research subjects are allowed compensation or valuable consideration for incurred non-financial burdens, the amount of financial recognition for the subject’s time, effort, and inconvenience must be reviewed to ensure that such compensation does not constitute an undue inducement.
Compensation for oocyte providers’ time, effort, and inconvenience, if permitted by local laws and human subjects review committees, should be reasonably consistent with compensation levels for other types of research participation involving similarly invasive and burdensome medical procedures. Compensation levels should aim to acknowledge oocyte providers’ non-financial burdens incurred as a result of their research participation, such as their physical discomfort and effort.
At no time should payments or other rewards of any kind be given for the number or quality of the oocytes that are to be provided for research.
Oocyte procurement must be performed only by medically qualified and experienced clinicians, and frequent monitoring and dose adjustment must be used to reduce the risk of ovarian hyperstimulation syndrome.
Due to the potential long-term effects of ovulation induction, women should undergo a limited number of hormonally induced ovarian stimulation cycles in a lifetime, regardless of whether they are induced for research or assisted reproduction. The limits should be determined by a thoughtful research review and oversight process, which should be informed by the latest available scientific information about the health risks.
A fertility clinic or other third party responsible for obtaining consent or collecting cells or tissues should not be paid for the material obtained. It should be eligible for specifically defined cost-based reimbursements and payments for professional services. Fertility clinics should not profit from providing tissues for research.
To help guide review committees through the ethical considerations surrounding oocyte collection and financial recognition of providers’ efforts, the ISSCR Ethics and Public Policy Committee developed an advisory report outlining their deliberations on these issues (Haimes et al., 2013).
-
This page assembles the sections of the Guidelines that specifically address the premature commercialization and unproven use of cellular products. Other sections of the Guidelines may be relevant to this issue (e.g., Chapter 4. Communications).
3.1 Classifying Stem Cell-, Cell-, and Tissue-based Interventions
Recommendation 3.1.1: Stem cells, cells, and tissues that are substantially manipulated or used in a non-homologous manner must be proven safe and effective for the intended use before being marketed to patients or incorporated into standard clinical care.
The therapeutic use of substantially manipulated stem cells, cells, or tissues or minimally manipulated stem cells, cells, or tissues for non-homologous treatments is complex, speculative, and has been shown to have risks to recipients. These products should be thoroughly tested in preclinical and clinical studies and evaluated by regulators as drugs, biologics, and advanced therapy medicinal products.
Minimally Manipulated Stem Cells, Cells, and Tissues
Minimally manipulated cells and tissues, such as, in some cases, fat tissue transferred from one part of the body to another, are generally subject to fewer regulatory requirements. When a stem cell-, cell-, or tissue-based intervention is claimed to be minimally manipulated and exempt from regulatory oversight on this basis, the responsibility rests on the clinician to invite independent scrutiny of their process of manipulation, such that scientific and regulatory experts can determine the proper level of regulatory oversight. When there is uncertainty or disagreement about the regulatory status of particular interventions, it is best to contact legally authorized regulatory bodies and seek their guidance concerning how specific interventions are classified. The US Food and Drug Administration, European Medicines Agency, Australian Therapeutic Goods Administration, Japanese Ministry of Health, Labour, and Welfare, and other regulators have released detailed standards to delineate when manipulation of cell-based products can no longer be considered minimal or their use homologous, and must therefore be subject to regulatory oversight as an advanced therapy product.
Substantially Manipulated Stem Cells, Cells, and Tissues
Substantially manipulated stem cells, cells, and tissues are subjected to processing steps that alter their original structural or biological characteristics. These processes can include isolation and purification processes, tissue culture and expansion of the cells, genetic manipulation, or other steps. For example, the extraction of cells from adipose tissue using enzymatic digestion, ultrasonic cavitation, or other means involves processing steps that can alter the original function of the cells imbedded in the tissue. The safety and efficacy profile of such an intervention needs to be determined for its particular indication using rigorous research methods. Safety and efficacy cannot be assumed because the composition of the intervention may differ from the original source tissue. Demonstration of safety and effectiveness will depend on the particular intervention and the specific condition targeted. Both to protect patients from risks and to help ensure that promising interventions are studied, it is critical that cells and tissues that have been substantially manipulated are evaluated by national regulators as drugs, biologics, and advanced therapy medicinal products.
Non-homologous Use of Stem Cells, Cells, and Tissues
Non-homologous use occurs when the stem cells, cells, or tissue are repurposed to perform a different basic function in the recipient than the cells or tissue originally performed prior to being removed, processed, and transplanted or otherwise delivered. For example, delivering adipose-derived stromal cells into the eye with the intent to treat macular degeneration would be a non-homologous use because the basic function of adipose tissue is not the trophic support of the retina. As with substantially manipulated cells and tissues, the non-homologous use of stem cells, cells, and tissues has potential benefits but can also pose serious risks. In the case of using adipose-derived stromal cells to treat macular degeneration, for example, there are well-documented reports of vision loss (Kuriyan et al., 2017). Such reports serve as a reminder that cells and tissues, depending on how they are administered, can cause serious harm. The benefit-risk ratio for non-homologous uses will depend on the particular intervention and the specific use. To protect patients from risks, and to ensure that necessary research is conducted, it is important that the safety and effectiveness of non-homologous uses be rigorously evaluated by regulators following the completion of well-designed and carefully controlled preclinical and clinical studies.
3.5 Unproven Stem Cell-based Interventions and Medical Innovation
The ISSCR condemns the administration of unproven stem cell- and other cell- and tissue-based interventions outside of the context of clinical research or medical innovation that is compliant with the guidelines in this document (see recommendation 3.5.2), particularly when it is performed as a business activity. Scientists and clinicians should not administer unproven interventions outside of clinical research or medical intervention as a matter of professional ethics. For the vast majority of medical conditions for which putative “stem cell therapies” or “regenerative therapies” are currently being marketed, there is insufficient evidence of safety and efficacy to justify routine or commercial use. Serious adverse events subsequent to such interventions have been reported and the long-term safety of most stem cell-, cord blood-, bone marrow-, and other cell-based interventions (i.e., mesenchymal stromal cells) remains undetermined. The premature commercialization of unproven stem cell interventions, and other cell-based interventions inaccurately marketed as “containing,” “acting on,” “derived from,” or “like” stem cells, not only puts patients at risk but also represents a serious threat to legitimate stem cell research. Widespread marketing and clinical use of unproven cell or tissue-based interventions reduces the number of individuals able to participate in credible clinical studies, risks jeopardizing the reputation of the field and causes widespread confusion about the actual state of scientific and clinical development.
Recommendation 3.5.1: The clinical use of unproven stem cell-based interventions should be limited to well-regulated clinical trials and medical innovations compliant with these guidelines (Recommendation 3.5.2) and local laws, policies, and regulations. Government authorities and professional organizations should establish and strictly enforce policies and regulations governing the commercial use of stem cell based medical interventions.
Historically, many medical innovations have been introduced into clinical practice without a formal clinical trials process. Some innovations have resulted in significant and long-lasting improvements in clinical care, while others have subsequently been demonstrated to be ineffective or harmful. Stem cell-based interventions typically entail complex manufacturing protocols that should rarely, if ever, be developed outside a formal clinical trials process. Nonetheless, in some very limited cases, clinicians may be justified in attempting medically innovative stem cell-based interventions in a small number of seriously ill patients. Although attempting medically innovative care is not research per se, it should not be embarked upon unilaterally. It is incumbent upon the clinician to obtain scrutiny by external experts through peer review, institutional oversight, and presentation of observations and data in peer-reviewed medical publication so that the knowledge can benefit all. Such limited attempts at medical innovation contrast with the advertisement, sale, and administration of unproven stem cell interventions.
Hospital Exemption
Regulators in some countries provide a “hospital exemption” to enable individualized care for patients. This exemption from requirements for regulatory evaluation of safety and effectiveness is only appropriate when the risks of the intervention are low and consistent with the risks typically associated with conventional surgical or medical procedures. Furthermore, the existence of such narrow exemptions should not be used as a vehicle for providing unapproved stem cell-based interventions or avoiding regulatory oversight, as occurs when cell-based interventions requiring pre-marketing authorization are inaccurately promoted as being exempt from regulatory scrutiny and approval requirements. Given the potentially serious risks associated with substantially manipulated tissues and cells and non-homologous uses, and the need to study their effectiveness, it is important that such interventions and uses be ineligible for these regulatory exemptions. In jurisdictions that provide hospital exemptions and have not established well-defined criteria that limit the scope of the exemption, regulators are urged to narrowly define them as including only low risk, minimally manipulated cells and tissues for homologous use.
Surgical Procedure Exemption
Regulators also often provide a narrow “same surgical procedure exemption,” excluding tissue- and cell-based interventions from certain regulatory requirements when cells or tissue are collected from, and delivered to, the same patient during the same procedure. These exemptions should be narrowly crafted to allow common surgical procedures like skin grafts, while excluding tissue and cell preparations that have been substantially manipulated or are being provided for a non-homologous use. This pathway should not be used to provide experimental and unapproved stem cell-based interventions.
Stem Cell Based Medical Innovation
Recommendation 3.5.2: Given the many uncertainties surrounding medical innovations involving stem cells and their direct derivatives, this pathway is rarely ethically and scientifically justifiable and should be limited to a very small number of patients and restricted to a) the off-label use of authorized therapies (see Recommendation 3.5.3), b) unproven interventions provided through expanded access pathways (see Recommendation 3.5.4), or c) minimally manipulated stem cell based interventions for homologous uses. Such interventions should only be provided to patients according to the highly restrictive provisions outlined in this section and the other referenced recommendations.
The written plan for the procedure must include:
Scientific rationale and justification explaining why the procedure has a reasonable chance of success, including any preclinical evidence of proof-of-principle for efficacy and safety.
Explanation of why the proposed stem cell-based intervention should be attempted rather than existing treatments.
Description of how the cells will be administered, including adjuvant drugs, agents, and surgical procedures.
Plan for clinical long-term follow-up and data collection to assess the effectiveness and adverse effects of the cell-based interventions.
The written plan is approved through a peer review process by appropriate experts who have no vested interest in the proposed procedure.
The written plan is approved by an independent oversight body after evaluating the risks and benefits for patients. In the academic context this would be routinely done through an institutional review process for human subjects research.
The patient is not eligible for an existing stem cell-based trial for this indication.
The clinical and administrative leadership of the healthcare institution supports the decision to attempt the medical innovation and the institution is held accountable for the innovative procedure.
All involved personnel have appropriate qualifications and training, and the institution where the procedure will be carried out has appropriate facilities and processes of peer review and clinical quality control monitoring.
Voluntary informed consent is provided by patients according to the ISSCR Informed Consent Standard (see Appendix 6).
There is an action plan for addressing adverse events that includes timely and adequate medical care and if necessary psychological support services.
Insurance coverage or other appropriate financial or medical resources are provided to patients to cover any adverse events arising from the intervention.
There is a commitment by clinician-scientists to use their experience with individual patients to contribute to generalizable knowledge. This includes:
Ascertaining outcomes in a systematic and objective manner
A plan for communicating outcomes, including negative outcomes and adverse events, to the scientific community to enable critical review (for example, as abstracts to professional meetings or publications in peer-reviewed journals). Initiating a formal clinical trial for the intervention in a timely manner after experience a very small number of patients.
Off-label Use
Recommendation 3.5.3 Off-label uses of stem cell-based interventions should be employed with particular care, given uncertainties often associated with off-label uses generally and associated with stem cell-based interventions specifically.
Physicians generally may use approved drugs and biologics for indications or patient populations other than those for which they have been shown to be safe and effective. This practice is commonly known as providing products on an “off-label” basis. Such off-label applications, distinct from administering products for the purposes for which they have been studied and approved, as specified on their prescribing information and package labels, constitute a common aspect of medical practice. Nevertheless, they present distinct challenges for stem cell, tissue or cell-based interventions.
First, depending on the jurisdiction, some stem cell-based interventions are not authorized for a specific use due to exemption from premarketing approval requirements. This can limit physicians’ access to reliable information on validated uses. Second, the complex biological properties of living cells and the limited clinical experience with cell-based therapies present uncertainties about long-term safety and effectiveness. Physicians should therefore exercise particular care when administering stem cell-based interventions on an off-label basis. As a rule, off-label use should only be offered when supported by high quality evidence or in situations consistent with current scientific knowledge, applicable regulations and institutional policies, and the standards of the international medical community. Patients must be informed in advance if a proposed off-label use has not been evaluated for safety and efficacy with respect to their specific medical condition. Off-label use of stem cell products is likely to increase as more stem cell therapies obtain pre-marketing authorization for particular indications. Providing such interventions on an off-label basis will need to be done with great caution, attentiveness to the available evidence base, and with the informed consent of potential recipients.
As a general principle, physicians should conduct controlled, supervised studies to establish safety and efficacy for new applications of products or interventions that have been approved in a distinct clinical setting. As evidence of safety and efficacy accumulates, regulatory bodies are provided with the data they require to consider expanding the indications that fall within the scope of product labeling.
Pre-approval Non-trial Access to Experimental Stem Cell-based Interventions
Recommendation 3.5.4 Pre-approval access to experimental stem cell-based interventions should be limited to well-regulated programs that require prior authorization from national regulators.
Patients understandably sometimes seek experimental interventions when there are no established and approved treatments for serious or terminal diseases and conditions. Regulatory authorization for pre-approval non-trial access programs (often described as “expanded access”) provides important checks and balances to ensure patient safety, facilitates drug development, and preserves the integrity of clinical trials. In particular, national regulatory bodies sometimes have access to important information about risks associated with particular investigational interventions that is not always available to individual patients or institutional review boards.
Substantial evidence to establish effectiveness for market approval
Recommendation 3.6.1.1 The introduction of novel products into routine clinical use should be dependent on the demonstration of substantial evidence of effectiveness in appropriately powered, well-controlled clinical trials, with statistically significant findings.
Regulatory approval for commercialization represents a key pivot point in a product’s translation. National governments and regulatory authorities should maintain rigorous review pathways to ensure that stem cell-based products conform to the highest standards of evidence-based medicine. Early interactions and advice during the product development process may support the accelerated development of safe and effective new therapies.
Even after clinical studies of the highest standard have demonstrated safety and efficacy and regulatory approval pathways have been cleared, close attention must be paid to ensuring the safety and effectiveness of interventions that have entered routine or commercial clinical use. Further, the fairness of access should be consistent with local legal requirements and standards and the standards of ethical, evidence-based medicine. These standards include ongoing monitoring of safety and outcomes and ensuring accessibility by those who have the most pressing clinical need.
Additional Resources
Addition context; papers written by members off the 2021 ISSCR Guidelines Steering Committee:
“Why stem cell guidelines needed an update: New criteria aim to reassure the public to permit progress in contentious research,” by Lovell-Badge (Nature)
“ISSCR Guidelines for Stem Cell Research and Clinical Translation: The 2021 Update,” by Lovell-Badge et al., ISSCR Guidelines Taskforce (Stem Cell Reports)
“Human Embryo Research, Stem Cell-derived Embryo Models and In Vitro Gametogenesis: Considerations Leading to the Revised ISSCR Guidelines,” Clark et al., (Stem Cell Reports)
“ISSCR Guidelines for the Transfer of Human Pluripotent Stem Cells and Their Direct Derivatives into Animal Hosts,” by Hyun et al. (Stem Cell Reports)
“ISSCR’s Guidelines for Stem Cell Research and Clinical Translation: Supporting the Development of Safe and Efficacious Stem Cell-Based Interventions,” by Turner (Stem Cell Reports)
"New Guidelines for Stem Cell and Embryo Research from the ISSCR," by Anthony et al. (Cell Stem Cell)
"ISSCR Guidelines Uphold Human Right to Science for Benefit of All," by Robin Lovell-Badge, Zubin Master, and Bartha Knoppers (Nature)
Visit The Archives for the previous versions of the ISSCR Guidelines for Stem Cell Research and Clinical Translation, as well as their translations.